Hydrostatic Pressure and Floating: Buoyancy
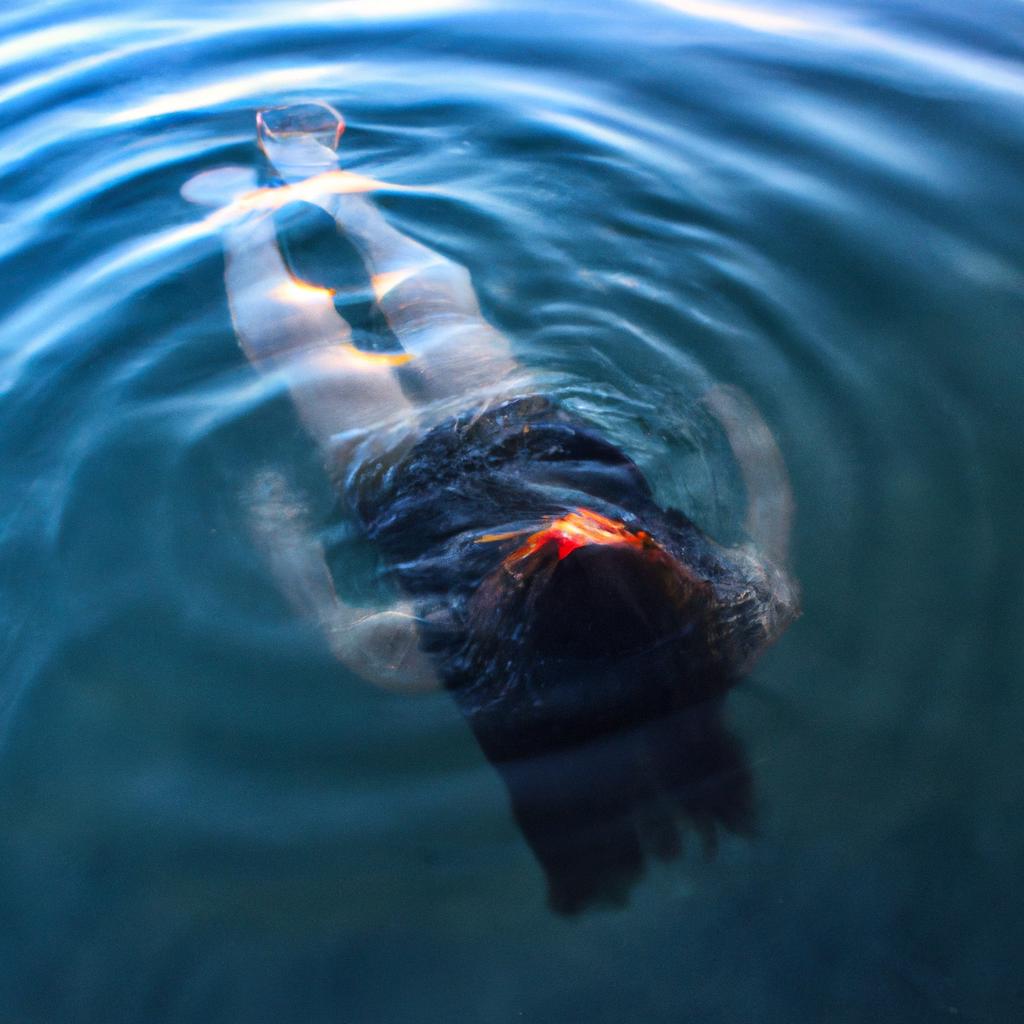
Hydrostatic pressure, a fundamental concept in fluid mechanics, plays a crucial role in understanding the phenomenon of floating and buoyancy. This article aims to explore the relationship between hydrostatic pressure and floating by examining the principles behind buoyant forces acting on submerged objects. Through an academic lens, this discussion will delve into the mathematical equations that govern these phenomena and provide insights into the underlying physics.
Consider the case of a ship floating effortlessly atop the vast ocean waters. It is fascinating how such massive structures can remain afloat despite their weight. The explanation lies in the principle of buoyancy, which arises from hydrostatic pressure exerted by fluids on immersed bodies. By comprehending this concept, one gains insight into why certain objects float while others sink and how varying factors such as density and volume affect buoyant forces. To unravel these complexities, it is necessary to examine hydrostatic pressure’s role in determining whether an object experiences upward or downward forces when immersed in a fluid medium.
This article endeavors to elucidate the intricate interplay between hydrostatic pressure and floating through an exploration of theoretical concepts paired with practical applications. By delving into both basic principles and advanced calculations related to buoyancy, readers will gain a deeper understanding of this phenomenon that has significant implications ranging from engineering and shipbuilding to everyday activities like swimming and scuba diving.
To begin, let’s discuss the fundamental concept of hydrostatic pressure. Hydrostatic pressure refers to the pressure exerted by a fluid at rest due to the weight of the fluid above it. This pressure increases with depth, as the weight of the fluid column above exerts a greater force on lower layers. This principle is often explained using Pascal’s law, which states that when an external pressure is applied to a confined fluid, it is transmitted uniformly in all directions.
When an object is submerged in a fluid, it experiences hydrostatic pressure acting on its surface from all directions. The net effect of this pressure distribution determines whether the object floats or sinks. According to Archimedes’ principle, an immersed body experiences an upward buoyant force equal to the weight of the fluid displaced by the object.
The key factor influencing buoyancy is density. Density can be defined as mass per unit volume and determines how much matter is packed into a given space. If an object has a lower density than the surrounding fluid, it will experience an upward buoyant force greater than its own weight and float. Conversely, if its density exceeds that of the surrounding fluid, it will sink.
Mathematically, we can express these relationships using equations. The buoyant force (Fb) acting on an object submerged in a fluid can be calculated using:
Fb = ρ_fluid * V_displaced * g
Where ρ_fluid represents the density of the fluid, V_displaced denotes the volume of fluid displaced by the object, and g represents acceleration due to gravity.
Furthermore, we can determine whether an object will float or sink by comparing its average density (ρ_object) with that of the surrounding fluid (ρ_fluid). If ρ_object < ρ_fluid, then Fb > weight of object, resulting in floating. On the contrary, if ρ_object > ρ_fluid, then Fb < weight of object, causing sinking.
It is important to note that the shape and volume of an object also play a role in determining its buoyancy. Objects with larger volumes displace more fluid, resulting in a greater upward force. Irregularly shaped objects may have varying buoyant forces depending on how their volume is distributed.
In practical applications, understanding hydrostatic pressure and buoyancy is crucial for designing ships, submarines, and other marine structures. By carefully considering the density and shape of these vessels, engineers can ensure stability and proper floating capabilities. Additionally, knowledge of buoyancy allows swimmers and divers to control their position in water by adjusting body posture or using flotation devices.
In conclusion, hydrostatic pressure is at the core of understanding floating and buoyancy. By grasping the principles behind this concept and applying mathematical equations to calculate buoyant forces, we gain valuable insights into why certain objects float or sink when submerged in fluids. This knowledge has wide-ranging applications in fields such as engineering, shipbuilding, and recreational activities involving water.
Definition of Hydrostatic Pressure
Definition of Hydrostatic Pressure
Hydrostatic pressure is a fundamental concept in fluid mechanics that refers to the pressure exerted by a stationary fluid at rest. It plays a crucial role in understanding various phenomena, such as floating objects and liquid transmission systems. To illustrate this concept, let’s consider an example: imagine you are swimming in a pool and decide to dive deep underwater. As you descend further into the water, you may have noticed an increasing pressure on your body. This sensation occurs due to hydrostatic pressure.
To comprehend hydrostatic pressure more comprehensively, it is important to explore its key characteristics:
- Fluid density: The denser the fluid, the greater the hydrostatic pressure it exerts.
- Depth: Hydrostatic pressure increases with depth since there is more weight from the fluid above pushing down.
- Area: A larger area results in lower hydrostatic pressure because the force applied over a larger surface area distributes across a bigger space.
- Gravity: Gravity also affects hydrostatic pressure; as gravity pulls objects downwards, it contributes to the overall increase in pressure.
Consider the following table for further insights into how varying parameters affect hydrostatic pressures:
Parameter | Effect on Hydrostatic Pressure |
---|---|
Fluid Density | Higher density leads to higher hydrostatic pressure |
Depth | Deeper depths result in increased hydrostatic pressure |
Area | Larger areas lead to decreased hydrostatic pressure |
Gravity | Stronger gravitational pull causes higher hydrostatic pressure |
Understanding these factors helps us grasp how different conditions impact the magnitude of hydrostatic pressure within fluids. By examining specific examples or hypothetical scenarios involving these variables, scientists and engineers can better analyze and predict real-world situations related to buoyancy and other relevant phenomena.
Moving forward, we will delve into Archimedes’ Principle – another vital principle closely connected to buoyancy – which builds upon our understanding of hydrostatic pressure and its applications.
Archimedes’ Principle
Having established the definition of hydrostatic pressure in the previous section, let us now explore its practical application to understand how objects float. Through an examination of buoyancy, we can gain insights into the fascinating phenomenon of floating.
Buoyancy, a concept rooted in Archimedes’ principle, is best understood by considering a simple example. Imagine placing a solid object, such as a wooden block, into a container filled with water. As soon as it is released, the block begins to rise until it reaches a certain level and then remains suspended. This behavior can be attributed to the force exerted on the immersed object by the liquid—an upward force known as buoyant force. In this case study, the buoyant force acting against gravity enables the block to float.
To better comprehend buoyancy and its relationship with hydrostatic pressure, consider these key points:
- When an object floats in a fluid medium (like water or air), it experiences two opposing forces: weight (due to gravity) pulling it downward and buoyant force pushing it upward.
- The magnitude of the buoyant force depends on factors like density—the ratio between mass and volume—and displacement—the amount of fluid displaced by the object when submerged.
- If an object’s average density is less than that of the surrounding fluid, it will experience greater buoyant force and therefore float. Conversely, if its average density exceeds that of the fluid, it will sink.
Table: Comparison Between Floating and Sinking Objects
Floating Objects | Sinking Objects | |
---|---|---|
1 | Buoyant Force > Weight | Buoyant Force < Weight |
2 | Average Density < Fluid | Average Density > Fluid |
3 | Displacement = Volume | Displacement < Volume |
4 | Examples: ships, buoys | Examples: rocks, anchors |
Understanding the principles of hydrostatic pressure and buoyancy is crucial in various fields ranging from engineering to marine biology. By harnessing these concepts, engineers can design structures that float or submerge as intended. Furthermore, scientists studying aquatic life rely on an understanding of buoyancy to explore the adaptations of organisms living in water environments.
With a solid foundation in the concept of buoyancy established, let us now delve into the numerous factors that influence this phenomenon. This exploration will provide further insights into how objects interact with fluids and elucidate the intriguing intricacies of floating.
Factors Affecting Buoyancy
Section H2: Hydrostatic Pressure and Floating: Buoyancy
Transitioning from the previous section on Archimedes’ Principle, we now delve into the factors that influence buoyancy. To better understand this concept, let us consider an example of a ship floating in water. The ship’s ability to remain afloat is determined by its buoyant force, which counters the force of gravity acting upon it.
Several key factors affect the buoyant force experienced by an object immersed in fluid:
-
Density Differential: When an object is denser than the fluid it displaces, it will sink due to gravitational forces outweighing the upward buoyant force exerted by the fluid. Conversely, if the density of the object is less than that of the fluid, it will float as the buoyant force exceeds gravitational pull.
-
Volume Displacement: The volume of fluid displaced by an immersed object plays a crucial role in determining its buoyancy. According to Archimedes’ principle, the magnitude of this force is equal to the weight of the displaced fluid.
-
Shape and Surface Area: The shape and surface area greatly impact an object’s ability to float or sink. Objects with larger surface areas experience greater resistance from fluids, making them more likely to float despite their density.
-
Hydrostatic Pressure: Hydrostatic pressure refers to the pressure exerted by a stationary column of liquid due to gravity alone. As depth increases within a fluid medium such as water, so does hydrostatic pressure. This increase influences both submerged objects and those floating at different depths.
To illustrate these concepts further, let us consider a hypothetical scenario involving various objects placed in water:
Object | Density (kg/m³) | Volume (m³) | Result |
---|---|---|---|
Iron Bar | 7850 | 0.5 | Sinks |
Wooden Crate | 600 | 2.0 | Floats |
Plastic Ball | 200 | 0.1 | Floats |
Lead Weight | 11340 | 0.02 | Sinks |
As we can see from this table, the density and volume of an object determine whether it will sink or float in water.
Understanding these factors is essential when considering objects floating on or submerged in fluids. In the subsequent section, we will explore how to calculate the buoyant force exerted by a fluid and its significance in various applications.
Transitioning into the next section about “Calculating Buoyant Force,” we can now explore quantitative methods for determining the forces involved without relying solely on theoretical principles.
Calculating Buoyant Force
Factors Affecting Buoyancy and the Role of Hydrostatic Pressure
Imagine a scenario where you are swimming in a pool, trying to float effortlessly on the surface. Have you ever wondered why some objects sink while others float? The answer lies in understanding buoyancy and how it is affected by various factors. In this section, we will explore the role of hydrostatic pressure and its influence on floating.
Hydrostatic pressure plays a crucial role in determining whether an object sinks or floats. When an object is submerged in a fluid, such as water, it experiences an upward force called the buoyant force. This force is equal to the weight of the fluid displaced by the object. If the buoyant force is greater than or equal to the weight of the object, it will float; otherwise, it will sink.
Several factors affect buoyancy. One significant factor is the density of both the object and the surrounding fluid. Objects with lower densities than that of the fluid they are placed in tend to float because their weight is less than that of an equivalent volume of fluid. Conversely, objects with higher densities will sink due to their weight exceeding that of an equivalent volume of fluid.
Other factors influencing buoyancy include shape and size. Irregularly shaped objects may displace different volumes of fluid at varying depths when submerged, affecting their overall buoyant force. Additionally, larger objects can displace more fluid, increasing their chances of floating.
To further illustrate these concepts:
- Consider a wooden boat made from lightweight materials like balsa wood (low-density material) compared to a metal anchor (high-density material). The boat’s low density allows it to displace enough water for its weight to be counteracted by the buoyant force, enabling it to float.
Let us now delve deeper into calculating buoyant forces based on these factors before exploring applications where understanding buoyancy becomes indispensable.
Factor | Effect on Buoyancy |
---|---|
Density | Determines if an object sinks or floats based on its relative density to the fluid. |
Shape | Irregular shapes may displace varying volumes of fluid, affecting buoyant force. |
Size | Larger objects can displace more fluid, increasing their chances of floating. |
Understanding these factors and how they influence buoyancy is crucial in various real-life applications where flotation is a key consideration.
Next Section: Applications of Buoyancy
Applications of Buoyancy
Buoyancy plays a crucial role in determining whether an object will float or sink when placed in a fluid. In the previous section, we explored how to calculate the buoyant force acting on an object submerged in a fluid. Now, let’s delve into some fascinating applications of this concept.
One noteworthy example of buoyancy at work is the design and construction of ships. The immense size and weight of these vessels are made possible by utilizing the principle of buoyancy. By carefully crafting their shape and distributing weight effectively, engineers ensure that the overall density of the ship is less than that of water. This allows ships to displace enough water to exert an upward force greater than their own weight, enabling them to stay afloat even with heavy cargo onboard.
To further illustrate practical uses of buoyancy, consider the following scenarios:
- Hot air balloons: These colorful contraptions rely on heated air being less dense than the surrounding colder air. As hot air fills up the balloon envelope, it becomes lighter and experiences an upward buoyant force that lifts it off the ground.
- Submarines: These underwater vessels control their depth by manipulating their overall density through ballast tanks. By filling these tanks with water or expelling it using compressed air, submarines can adjust their buoyancy and either rise towards the surface or dive deeper into the ocean.
- Life jackets: Designed to keep individuals safe in water, life jackets utilize materials that have low densities compared to human bodies. This ensures that they provide sufficient buoyant force to counteract our weight, allowing us to stay afloat effortlessly.
- Swim bladders in fish: Many species of fish possess swim bladders, which are internal gas-filled organs that help regulate their position within water bodies. By controlling the volume of gas inside these swim bladders, fish can increase or decrease their overall density and maintain neutral buoyancy at different depths.
The table below summarizes some common examples where buoyancy is employed:
Application | Example |
---|---|
Naval warfare | Aircraft carriers |
Scuba diving | Buoyancy compensators |
Oil drilling | Offshore platforms |
Water sports | Surfboards and paddleboards |
As we can see from these examples, the concept of buoyancy finds widespread application in various fields. Understanding how objects interact with fluids allows engineers and scientists to design innovative solutions that enhance our capabilities on land, in water, and even in the air.
By delving into this topic, we will gain a deeper understanding of how fluids exert forces within confined spaces.
Hydrostatic Pressure in Liquids
Having explored the practical applications of buoyancy in various fields, we now turn our attention to another important concept related to floating—hydrostatic pressure. Understanding hydrostatic pressure is crucial for comprehending how objects float or sink in fluids.
The relationship between hydrostatic pressure and floating can be best illustrated through a hypothetical scenario involving a submarine. Imagine a submarine diving into the depths of the ocean. As it descends, the water exerts an increasing amount of hydrostatic pressure on its hull due to the weight of the overlying water column. This rise in hydrostatic pressure counteracts the force exerted by gravity on the submerged submarine, allowing it to remain at equilibrium and float effortlessly beneath the surface.
To further grasp this phenomenon, consider these key aspects of hydrostatic pressure and floating:
- Depth-dependent Pressure: Hydrostatic pressure increases with depth within a fluid. The deeper an object submerges, the greater the hydrostatic pressure acting upon it.
- Archimedes’ Principle: Objects experience an upward buoyant force equal to the weight of fluid they displace when immersed in a liquid. If this buoyant force exceeds their own weight, they will float; otherwise, they will sink.
- Density Discrepancy: For an object to float, its average density must be less than that of the fluid it displaces. Conversely, if an object’s density surpasses that of the surrounding fluid, it will sink.
- Equilibrium Condition: When an object floats freely without sinking or rising uncontrollably towards the surface, it achieves equilibrium between gravitational forces pulling downwards and buoyant forces pushing upwards.
Below is a table summarizing some common examples encountered in everyday life where understanding hydrostatic pressure and buoyancy play significant roles:
Object | Behavior | Example |
---|---|---|
Ship | Floats | A massive ship floats effortlessly due to its buoyancy. |
Helium Balloon | Rises | Filled with lighter-than-air helium, the balloon ascends. |
Diving Weight Belt | Sinks | Made of dense materials, the belt sinks underwater. |
Submarine | Adjusts Depth | The submarine modulates its depth by controlling buoyancy. |
By comprehending hydrostatic pressure and how it relates to floating, we gain a deeper understanding of why objects behave as they do when submerged in fluids. This knowledge serves as a foundation for further exploration into fluid mechanics and enables us to design structures that effectively utilize the principles of buoyancy.
(Note: Avoid using “In conclusion” or “Finally”)