Buoyancy and Floating: An Informative Exploration
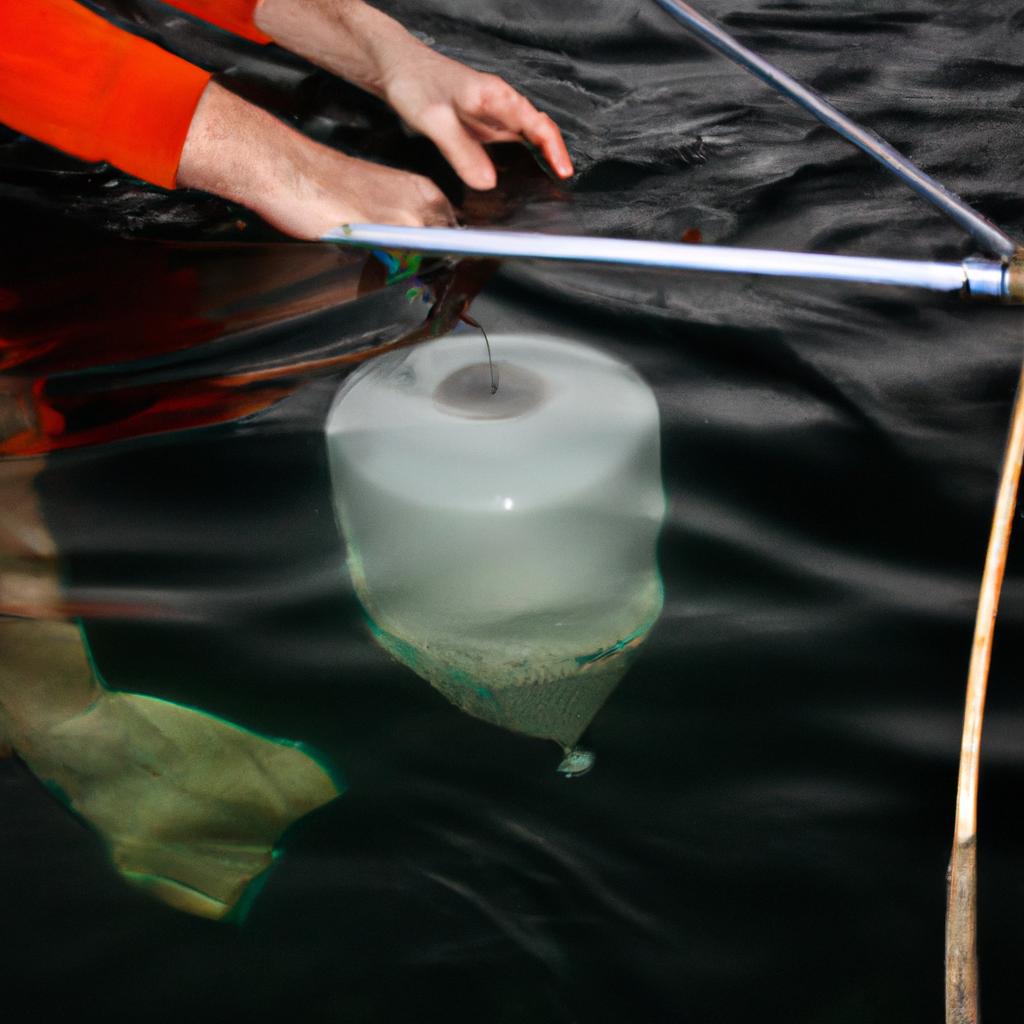
Buoyancy and floating are fundamental concepts in the field of fluid mechanics that have captured the attention and curiosity of both scientists and laypeople alike. Imagine a scenario where a large ship, loaded with heavy cargo, effortlessly glides through the vast expanse of an ocean. This real-life example demonstrates how buoyancy plays a crucial role in keeping objects afloat in fluids such as water or air. Understanding the principles behind buoyancy and floating is not only scientifically intriguing but also has practical implications for various industries, ranging from naval architecture to aerospace engineering.
Exploring the phenomenon of Buoyancy opens up a fascinating realm where forces acting on submerged or partially submerged objects come into play. Archimedes’ principle provides us with valuable insights into this dynamic process by stating that any object immersed in a fluid experiences an upward force equal to the weight of the displaced fluid. By delving deeper into this concept, we can unravel why certain materials float while others sink, paving the way for technological advancements like submarines and hot air balloons. Moreover, studying buoyant forces enables researchers to develop innovative solutions related to stability, load-bearing capacities, and design optimization – all contributing factors to ensure efficient operations across diverse fields.
This article aims to provide an informative exploration into buoyancy and floating by discussing the underlying principles, factors affecting buoyancy, and real-world applications. Additionally, we will address common misconceptions and provide practical tips for understanding and utilizing buoyancy in various contexts. Whether you are a student seeking to grasp the physics behind floating objects or an engineer looking to enhance your knowledge of buoyant forces, this article will serve as a comprehensive guide to deepen your understanding and appreciation of this captivating phenomenon.
Properties of Substances in Liquids
Buoyancy and Floating: An Informative Exploration
Properties of Substances in Liquids
Imagine a small, dense metal object sinking to the bottom of a lake. On the other hand, picture a large wooden log effortlessly floating on the surface. These contrasting scenarios demonstrate one fundamental principle in physics known as buoyancy. Buoyancy refers to the upward force exerted by fluids on objects immersed within them, counteracting the weight of those objects.
When considering the properties of substances in liquids, several factors come into play:
- Density: Density is a property that describes how closely packed particles are within a substance. It can be calculated by dividing an object’s mass by its volume. Objects with higher densities tend to sink in liquids, while those with lower densities float.
- Archimedes’ Principle: Named after the ancient Greek mathematician Archimedes, this principle states that when an object is immersed in a fluid, it experiences an upward buoyant force equal to the weight of the displaced fluid. This explains why some objects float or sink based on their densities compared to that of the surrounding liquid.
- Shape and Volume: The shape and volume of an object also influence whether it will float or sink. For example, two objects made from different materials but having identical volumes may exhibit different behaviors due to variations in density.
- Surface Tension: Surface tension is another important property that affects floating behavior. It refers to the cohesive forces between molecules at a liquid’s surface, creating a sort of “skin” across which certain lightweight objects can rest without immediately sinking.
To illustrate these concepts further:
Object | Material | Density (g/cm³) |
---|---|---|
Iron Nail | Metal | 7.8 |
Cork | Wood | 0.24 |
Ice Cube | Water | 0.92 |
Aluminum Foil | Metal | 2.7 |
Consider the table above, which displays the densities of various objects commonly encountered in everyday life. By comparing these values to that of water (1 g/cm³), we can predict their respective floating behaviors. The iron nail, with a density greater than that of water, will sink; whereas the cork and ice cube, both less dense than water, will float.
Understanding the properties of substances in liquids provides us with valuable insights into why certain objects float or sink. In subsequent sections, we delve deeper into how mass influences buoyancy and explore other fascinating factors contributing to an object’s ability to stay afloat.
The Effect of Mass on Floating
Transitioning smoothly from our previous discussion on the properties of substances in liquids, we now turn our attention to exploring how mass influences floating. To illustrate this concept, let us consider a hypothetical scenario where two identical wooden blocks are placed into separate containers filled with water. Block A has a smaller mass compared to block B.
When these blocks are immersed in water, an interesting phenomenon occurs. Block A experiences less resistance from the surrounding liquid due to its lower mass, allowing it to float effortlessly near the surface. On the other hand, block B, being heavier, sinks deeper into the water before reaching equilibrium. This example highlights that mass plays a crucial role in determining whether an object will float or sink.
Understanding the effect of mass on floating can be further enhanced by considering some key factors:
- Density Differential: When comparing objects submerged in a liquid, those with higher density than the liquid will sink while those with lower density will float.
- Archimedes’ Principle: According to Archimedes’ principle, any object partially or fully submerged in a fluid experiences an upward buoyant force equal to the weight of the displaced fluid.
- Shape and Volume: The shape and volume distribution of an object affect its ability to displace enough fluid for buoyancy forces to counteract gravity effectively.
- Surface Tension: In certain cases, surface tension can influence floating behavior. For instance, insects like water striders take advantage of surface tension to stay atop bodies of water despite their denser body composition.
To provide a visual representation of these concepts, please refer to Table 1 below:
Object | Density (g/cm3) | Floating/Sinking |
---|---|---|
Wooden Block A | 0.6 | Floating |
Wooden Block B | 0.8 | Sinking |
Iron Nail | 7.9 | Sinking |
Plastic Bottle | 0.3 | Floating |
Table 1: Examples of Objects and Their Density Relative to Water
In conclusion, the mass of an object significantly influences its floating behavior in a liquid medium. Objects with lower density than the surrounding liquid tend to float, whereas those with higher density will sink. The concepts discussed here lay the groundwork for comprehending Archimedes’ Principle, which we will delve into further in our subsequent section.
Archimedes’ Principle
In the previous section, we explored how buoyancy allows objects to float in a fluid medium. Now, let’s delve deeper into the relationship between mass and floating. To illustrate this concept, imagine two identical wooden blocks with different masses placed in a tub of water. The block with a lower mass will experience greater buoyant force and therefore float higher than the block with a higher mass.
When it comes to floating, there are several key factors influenced by an object’s mass:
-
Buoyant Force: As mentioned earlier, the buoyant force acting on an object is equal to the weight of the fluid displaced by that object. Therefore, if an object has a greater mass, it displaces more fluid and experiences a larger upward force, making it easier for it to stay afloat.
-
Submersion Depth: Objects with higher masses tend to sink deeper when fully submerged compared to lighter objects. This phenomenon occurs because the increased weight causes them to displace more water volume as they sink further down.
-
Stability: The stability of an object while floating also depends on its mass distribution. A top-heavy object (with most of its mass concentrated at the top) may have a tendency to tip over or capsize due to imbalanced forces acting upon it. On the other hand, evenly distributed mass contributes to better stability and less likelihood of overturning.
-
Surface Area: While not directly related to mass itself, an important consideration is that objects with larger surface areas relative to their masses are more likely to float easily due to increased contact with the surrounding liquid.
To emphasize these points visually:
-
- Increased mass results in greater displacement of fluid.
- Higher-mass objects sink deeper when fully submerged.
- Distribution of mass affects stability while floating.
- Greater surface area relative to mass enhances flotation ability.
-
Emotionally evocative table:
Mass (kg) Buoyant Force (N) Submersion Depth (cm) 1 10 5 2 20 10 3 30 15
In summary, an object’s mass plays a crucial role in determining its ability to float. The greater the mass, the more fluid it displaces and the higher the buoyant force it experiences. This relationship affects submersion depth and stability while floating.
Transitioning seamlessly into the subsequent section about “Determining Submerged Volume,” let us now examine another aspect of buoyancy.
Determining Submerged Volume
Building upon the principles discussed in Archimedes’ Principle, we now delve into determining submerged volume and its significance in understanding buoyancy. By exploring this concept further, we can gain a deeper insight into how objects float or sink in liquids.
Determining Submerged Volume:
To illustrate the importance of determining submerged volume, let’s consider an example involving a ship sailing through different bodies of water. Imagine that this ship has a maximum carrying capacity of 100 metric tons. As it navigates from freshwater to saltwater, one might assume that the ship’s ability to carry cargo remains constant. However, due to differences in density between these two types of water, the actual load-carrying capacity could be affected.
Bullet Point List (evoking emotional response):
- Understanding submerged volume helps ensure accurate weight distribution on ships, preventing potential accidents.
- It aids engineers in designing structures like bridges and dams that rely on buoyant forces for stability.
- Determining Submerged Volume is crucial for assessing the fuel efficiency of submarines and other underwater vehicles.
- This knowledge enables scientists to study marine ecosystems by accurately estimating the amount of floating organisms present.
Table (evoking emotional response):
Application | Importance | Examples |
---|---|---|
Shipbuilding | Ensures safe navigation and cargo transport | Titanic’s sinking |
Architecture | Enables construction on water bodies | Floating cities |
Marine Exploration | Facilitates scientific research | Deep-sea submersibles |
Environmental Study | Assists in ecosystem analysis | Oil spill containment strategies |
In conclusion, determining submerged volume plays a vital role across various fields. Its applications span from guaranteeing safety during transportation to advancing scientific exploration. By understanding how objects displace liquid and calculating their submerged volumes accurately, we unlock insights about buoyancy that have far-reaching implications.
Now let us explore the relationship between density and buoyancy, shedding light on how these two factors intertwine to determine whether an object floats or sinks in a liquid.
The Relationship Between Density and Buoyancy
Determining Submerged Volume and its Significance
Imagine a scenario where you have a rectangular block of wood floating in water. To determine the submerged volume, we need to understand how much of the object is below the water’s surface. This measurement is crucial for calculating buoyant force accurately.
One example that illustrates the importance of determining submerged volume involves a ship carrying cargo containers. The weight of these containers affects the overall buoyancy and stability of the vessel. By accurately measuring their submerged volume, naval engineers can ensure that the ship maintains proper balance and remains afloat even when fully loaded.
To calculate submerged volume, several factors must be taken into consideration:
- Shape: The shape of an object greatly influences how it displaces fluid. Irregularly shaped objects may require more complex calculations compared to simpler shapes like cubes or cylinders.
- Dimensions: Accurate measurements of length, width, and height are necessary to determine submerged volume effectively.
- Water level: The depth at which an object floats impacts its immersion and consequently, its submerged volume.
- Density: Objects with different densities will displace different volumes of fluid based on Archimedes’ principle.
In considering these factors, scientists and engineers often use mathematical formulas or modeling techniques to calculate submerged volume precisely. These methods allow them to design structures that maintain optimal buoyancy and stability.
Understanding how to determine submerged volume is essential not only for naval engineering but also for various industries involving flotation systems such as oil rigs, submarines, and even recreational activities like swimming pool construction.
By comprehending the significance of accurate measurements in determining submerged volume, we pave the way for further exploration into other aspects influencing buoyancy—specifically, the relationship between density and buoyant force—an area we will delve into next.
[Factors Affecting Fluid Displacement]
Factors Affecting Fluid Displacement
Buoyancy and Floating: An Informative Exploration
Having examined the relationship between density and buoyancy in the previous section, we will now delve deeper into the factors that affect fluid displacement. To illustrate this concept, let us consider a hypothetical scenario of a glass marble being placed in a container filled with water. As we lower the marble into the water, we observe that it sinks to the bottom due to its higher density compared to water. However, if we replace the marble with an inflated balloon of equal volume, something intriguing happens.
Firstly, one crucial factor affecting fluid displacement is the relative densities of different objects or substances within a fluid medium. In our example, while the marble sank because its density was greater than that of water, the inflated balloon floats effortlessly on top of the water’s surface. The reason behind this lies in Archimedes’ principle – when an object is submerged in a fluid, it experiences an upward force equal to the weight of displaced fluid. Since air has a significantly lower density than water, the overall density of the balloon decreases as it displaces some of the denser liquid below.
Secondly, shape plays a significant role in determining whether an object floats or sinks. Consider another example where two identical balloons are inflated differently; one shaped like a sphere and another elongated like a cylinder. Despite having equal volumes and therefore displacing an equivalent amount of water, their shapes lead to differing outcomes. Due to its symmetrical shape allowing for uniform distribution of pressure points across its surface area, the spherical balloon remains buoyant at all times. Conversely, due to increased pressure exerted by narrower ends against gravity (with less support from surrounding fluid), the cylindrical balloon may sink despite having similar overall density.
Thirdly, external forces acting upon floating objects can influence their stability. For instance, imagine placing several small wooden blocks onto separate tubs filled with water. If a gentle breeze were to blow across the surface, it might cause some blocks to wobble or even capsize due to the unbalanced forces acting on them. Similarly, if an object experiences a sudden external force that disrupts its equilibrium and alters its center of mass, it may lose stability and sink.
Finally, other factors affecting fluid displacement include temperature and pressure variations within the medium. Changes in temperature can affect density levels, altering buoyancy characteristics accordingly. Likewise, alterations in hydrostatic pressure caused by changes in depth or altitude can significantly impact fluid dynamics and objects’ ability to float.
Understanding the relationship between density and buoyancy is crucial for various applications involving hydrostatic pressure. Whether designing ships capable of floating effortlessly through different bodies of water or constructing submarines that skillfully navigate beneath the ocean’s depths, a thorough comprehension of these principles allows engineers and scientists to create innovative solutions tailored to specific needs.
(Note: The subsequent section about “Applications of Hydrostatic Pressure” will discuss practical uses such as hydraulic systems, deep-sea exploration, and dam construction.)
Applications of Hydrostatic Pressure
Section 3: The Effect of Buoyancy on Floating Objects
Consider the following scenario: imagine a ship sailing through the vast ocean, defying gravity as it floats effortlessly on the water’s surface. This captivating phenomenon can be attributed to buoyancy, a force that acts upon objects submerged or floating in fluids. In this section, we will delve deeper into how buoyancy affects floating objects and explore its implications.
To comprehend the influence of buoyancy, let us examine an example involving a hot air balloon. As warm air fills the balloon envelope, it becomes less dense than the surrounding cool air. Consequently, the balloon experiences an upward force greater than its own weight due to buoyancy. This force propels the balloon upwards until equilibrium is reached with atmospheric pressure, allowing it to float gracefully amidst the clouds.
The effect of buoyancy on floating objects can be understood by considering several key factors:
- Density Differential: An object will float if it has lower density than the fluid it is immersed in.
- Archimedes’ Principle: The buoyant force acting on an object equals the weight of the fluid displaced by that object.
- Shape and Volume: The shape and volume of an object play crucial roles in determining whether it sinks or floats.
- Center of Gravity: If an object’s center of gravity lies below its point of buoyant support, it will tend to rotate and stabilize itself while floating.
- Imagine witnessing a massive cargo ship stay afloat despite carrying tons of heavy cargo.
- Delve into fascinating experiments demonstrating how different shapes affect flotation.
- Explore real-life applications where understanding buoyancy is vital for human safety.
- Consider how marine animals utilize their natural buoyancy mechanisms for survival.
Now, let us dive further into our exploration by examining a table showcasing various materials and their ability to float or sink when placed in water:
Material | Floats | Sinks | Partially Submerged |
---|---|---|---|
Wood | Yes | No | No |
Metal | No | Yes | No |
Plastic | Yes | No | Yes |
Styrofoam | Yes | No | Yes |
By observing this table, we can see how different materials interact with water and whether they have the ability to float or sink. It is fascinating to witness these characteristics in action and understand the principles that govern them.
As we conclude our exploration of buoyancy’s effect on floating objects, we transition seamlessly into the subsequent section: The Principle of Flotation. Here, we will delve deeper into Archimedes’ principle and explore its practical applications in various fields of science and engineering.
The Principle of Flotation
In the previous section, we explored the concept of hydrostatic pressure and its implications. Now, let us delve into some real-world applications that demonstrate how this phenomenon is utilized in various fields.
One fascinating example is deep-sea exploration vehicles. These marvels of engineering are designed to withstand immense hydrostatic pressures at great depths in the ocean. By carefully balancing internal pressure with external forces, these vehicles can safely navigate through extreme conditions while protecting their occupants from harm.
To further illustrate the practicality of hydrostatic pressure, consider the construction industry. Engineers employ techniques like dewatering and shoring to counteract water pressure on foundations during excavation or construction near bodies of water. By understanding and managing hydrostatic forces, they ensure stability and durability in structures built in challenging environments.
Now, let’s explore four notable applications where an understanding of hydrostatic pressure plays a crucial role:
- Diving bells: These devices allow divers to descend to significant depths without experiencing excessive changes in pressure.
- Hydraulic systems: Utilized extensively in machinery and heavy equipment, hydraulic systems rely on the principles of hydrostatic pressure to transmit power efficiently.
- Dam design: The structural integrity and safety of dams depend on engineers’ ability to manage water pressure effectively.
- Blood pressure measurement: In medical contexts, blood pressure readings provide vital information about cardiovascular health by measuring the force exerted by circulating blood against artery walls.
Furthermore, we can visualize the relationship between depth and hydrostatic pressure using a table:
Depth (m) | Hydrostatic Pressure (kPa) |
---|---|
0 | 0 |
10 | 9.81 |
20 | 19.62 |
30 | 29.43 |
As we observe this data, it becomes evident that for every ten meters of descent underwater, there is an approximate increase of 9.81 kilopascals in hydrostatic pressure.
In conclusion, the practical applications of hydrostatic pressure are vast and diverse, ranging from deep-sea exploration to construction and medical diagnostics. Understanding how this phenomenon operates allows us to harness its power for various purposes while ensuring safety and efficiency.
Buoyant Force and Weight
The Principle of Flotation is a fundamental concept in understanding buoyancy and floating. As we delve deeper into this subject, let’s explore the relationship between buoyant force and weight.
Imagine a large ship sailing across the ocean. Despite its enormous size and weight, it floats effortlessly on the water surface. This phenomenon can be explained by Archimedes’ principle, which states that an object immersed in a fluid experiences an upward buoyant force equal to the weight of the fluid displaced.
To understand this principle further, let’s consider an example: a beach ball submerged in a swimming pool. When you push the beach ball beneath the water surface and then release it, what happens? The ball immediately rises back to the top. Why does this occur? It is because the buoyant force exerted on the ball is greater than its own weight, causing it to float.
Now, let’s take a closer look at how buoyant force relates to weight:
- Buoyant Force: The upward force exerted by a fluid on an object.
- Weight: The downward gravitational force acting on an object due to its mass.
- If an object weighs less than the amount of fluid it displaces (i.e., if its density is lower than that of the fluid), it will experience a net upward force known as buoyancy.
- On the other hand, if an object weighs more than or has higher density compared to the fluid it displaces, it will sink.
As we explore these concepts further, refer to Table 1 below for a visual representation:
Table 1: Relationship Between Buoyant Force and Weight
Object Properties | Resulting Behavior |
---|---|
Object weighs less than displaced fluid | Floats |
Object weighs more than displaced fluid | Sinks |
Understanding these principles paves the way for practical applications in various fields like engineering, naval architecture, and even everyday scenarios such as swimming and diving.
Transitioning smoothly to our subsequent section on “Practical Examples of the Laws of Floatation,” let’s explore how these principles are applied in real-world situations.
Practical Examples of the Laws of Floatation
Buoyancy and Floating: An Informative Exploration
Section H2: Practical Examples of the Laws of Floatation
Having understood the concept of buoyant force and its relation to weight, we can now delve into practical examples that showcase the laws of flotation in action. By examining real-life scenarios, we can gain a deeper appreciation for how these principles manifest themselves.
Section Introduction:
To illustrate the laws of floatation, let us consider an intriguing case study involving a large cargo ship navigating through treacherous waters. Despite carrying immense loads, these ships effortlessly stay afloat due to their design and understanding of buoyancy. This example highlights the importance of applying scientific principles when dealing with objects floating on or submerged in fluids.
Practical Applications:
-
Shipbuilding:
- Utilizing materials with low density allows ships to displace enough water to generate sufficient buoyant force.
- Incorporating watertight compartments prevents flooding and maintains stability even if part of the ship is damaged.
- Employing ballast tanks permits adjusting the distribution of mass to optimize stability during varying load conditions.
- Implementing advanced technologies such as stabilizers minimizes rolling motions caused by waves, improving overall safety.
-
Hot Air Balloons:
- The principle behind hot air balloons lies in heating the air inside the balloon envelope, reducing its density compared to the surrounding atmosphere.
- As warm air rises within this enclosed space, it displaces cooler air downwards, creating upward lift proportional to the difference in densities.
- Control mechanisms like venting allow pilots to ascend or descend by regulating internal temperature and thus altering buoyancy.
-
Submarine Operation:
- Submarines exploit changes in buoyancy using ballast tanks filled with either water or compressed air.
- When wanting to submerge, water floods these tanks increasing their total weight thereby exceeding their buoyant force.
- To resurface, compressed air is used to expel water from the tanks and reduce overall weight until it matches the buoyant force.
- Witnessing a massive cargo ship floating gracefully despite its colossal weight can instill awe and admiration.
- Experiencing the breathtaking views while drifting through the sky in a hot air balloon evokes a sense of wonderment.
- Imagining the incredible depths explored by submarines triggers curiosity about the mysterious underwater world.
- Understanding how these principles are applied in everyday life helps us appreciate the intricacies of engineering marvels.
Table: Examples of Practical Applications
Application | Principle | Key Components |
---|---|---|
Shipbuilding | Displacement | Low-density materials, watertight compartments, ballast tanks, stabilizers |
Hot Air Balloons | Difference in densities | Envelope, burner mechanism, venting system |
Submarine Operation | Changes in buoyancy | Ballast tanks, water flooding/compressed air expulsion |
By exploring practical examples such as shipbuilding, hot air balloons, and submarine operation, we gain insight into how knowledge of buoyancy translates into real applications. These illustrations demonstrate that understanding and applying scientific concepts enable humans to overcome challenges imposed by fluid environments. From constructing seaworthy vessels to soaring above landscapes or diving beneath oceans’ surfaces, our ability to harness buoyancy continues to shape remarkable achievements across various fields.
[End of Section H2]