Fluid Mechanics in Floating: The Dynamics
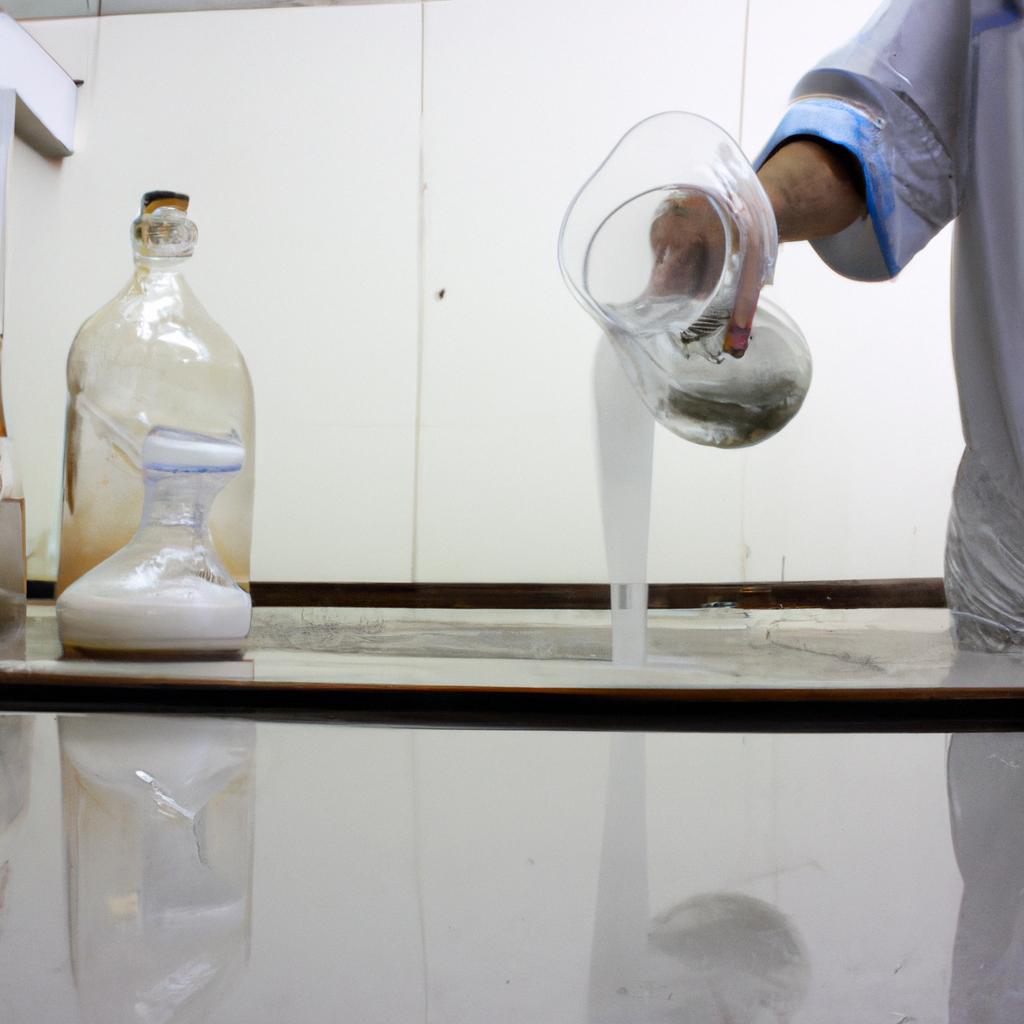
Fluid mechanics plays a crucial role in understanding the dynamics of floating objects. Whether it is a ship navigating through rough seas or a simple toy boat drifting on a calm pond, the behavior and stability of these floating bodies are governed by the principles of fluid mechanics. For instance, consider the case study of an oil tanker sailing across turbulent waters. The ability of such massive vessels to stay afloat amidst powerful waves relies heavily on the intricate interplay between various fluid forces acting upon them.
The study of fluid mechanics in the context of floating objects encompasses several key aspects. Firstly, buoyancy – the upward force exerted by a fluid on any object immersed within it – determines whether an object will float or sink. Through Archimedes’ principle, scientists have unraveled how this force depends on factors like volume displacement and density difference between the object and its surrounding medium. Additionally, hydrostatic pressure plays a significant role in determining equilibrium conditions for floating bodies, as variations in pressure distribution affect their overall stability.
In exploring these fundamental concepts and their practical applications, this article aims to shed light on the complex dynamics involved in floating systems. By delving into real-life examples and hypothetical scenarios, we can gain deeper insights into how different forces interact within fluids to influence the behavior of floating objects.
One example of the interaction between fluid forces and floating objects is the stability analysis of ships. Ships are designed to withstand various environmental conditions, including waves, wind, and currents. The shape and size of a ship’s hull are carefully engineered to maximize buoyancy and minimize drag. By understanding the principles of fluid mechanics, naval architects can optimize these designs to ensure that ships remain stable and maneuverable in different operating conditions.
Another interesting application is the study of hydrodynamics in sports such as swimming and diving. Athletes often use specialized techniques to minimize drag and maximize propulsion through the water. Understanding fluid mechanics allows coaches and athletes to refine their techniques for better performance.
Additionally, fluid mechanics plays a crucial role in offshore engineering, particularly in the design of oil platforms and floating wind turbines. These structures must be able to withstand strong ocean currents, waves, and wind loads while maintaining stability. By analyzing fluid flow patterns around these structures, engineers can optimize their designs for improved safety and efficiency.
In summary, fluid mechanics provides invaluable insights into the behavior of floating objects. From understanding buoyancy and hydrostatic pressure to optimizing designs for stability and performance, this field enables us to navigate complex aquatic environments safely and efficiently.
Understanding the principle of fluid dynamics
Understanding the Principle of Fluid Dynamics
Fluid dynamics is a branch of physics that focuses on studying the behavior and motion of fluids, such as liquids and gases. It provides insights into various phenomena, including how objects float in fluids. To comprehend this principle, let us consider the example of a ship floating on water. By examining the forces at play and exploring fundamental fluid dynamics concepts, we can gain valuable insight into this fascinating phenomenon.
In order to understand the principles governing fluid dynamics, it is essential to recognize the role played by different forces. When an object floats in a fluid like water, there are two primary forces acting upon it: buoyancy and gravity. Buoyancy force arises due to the pressure difference between the top and bottom surfaces of an immersed object. This force counteracts the weight of the object, allowing it to stay afloat. On the other hand, gravity pulls down on the object with a force equal to its weight.
To evoke an emotional response from readers when considering these forces, imagine standing on a beach witnessing waves crashing against rocks or observing colorful fish swimming effortlessly through coral reefs beneath crystal clear waters. These experiences remind us of nature’s awe-inspiring power and beauty—fluid dynamics plays an integral part in creating these mesmerizing scenes.
The understanding of fluid dynamics also relies heavily on key principles that help explain observations related to floating objects. Here are some important factors to consider:
- The density of an object determines whether it will sink or float in a particular fluid.
- Archimedes’ principle states that any body submerged in a fluid experiences an upward buoyant force equal to the weight of displaced fluid.
- Pascal’s law highlights how changes in pressure within confined fluids lead to transmission throughout their entirety.
- Bernoulli’s principle explains how variations in velocity result in corresponding changes in pressure along streamline flow paths.
Embracing these principles allows scientists and engineers not only to analyze current scenarios but also predict and model future fluid dynamics phenomena. By utilizing tables, such as the one presented below, researchers can organize pertinent data to enhance their understanding of fluid behavior.
Principle | Description | Application |
---|---|---|
Density | Measure of mass per unit volume | Determines if an object will sink or float |
Archimedes’ Law | The buoyant force on a body immersed in a fluid is equal to the weight of the displaced fluid | Predicts whether an object will float |
Pascal’s Law | Pressure applied to a confined fluid is transmitted undiminished throughout the entire fluid | Helps in designing hydraulic systems |
Bernoulli’s Law | As the speed of a moving fluid increases, its pressure decreases | Explains lift generated by airplane wings |
In summary, comprehending fluid dynamics is crucial for understanding floating objects. Through analyzing forces like buoyancy and gravity, along with principles such as density and pressure transmission, we gain insights into why certain objects float while others sink. In the subsequent section about “The role of pressure in fluid motion,” we delve deeper into how pressure influences various aspects of fluid behavior.
The role of pressure in fluid motion
Understanding the principle of fluid dynamics has laid a solid foundation for comprehending the intricate dynamics involved in floating. Building upon this knowledge, we now delve into the role of pressure in fluid motion, which plays a crucial part in determining how objects float and move within fluids.
To illustrate this concept, let us consider the case of a ship floating on water. When a ship is placed in water, it displaces an amount of water equal to its weight. This displacement creates an upward force known as buoyancy, acting against the downward force of gravity. The ability of the ship to remain afloat depends on whether these forces are balanced or not.
Pressure, defined as force per unit area, exerts its influence on floating objects by acting uniformly in all directions. In our example, the pressure beneath the ship’s hull is higher than above it due to the greater depth below the surface. This difference in pressure generates an upward force that helps keep the vessel buoyant.
Examining further into fluid mechanics, several key points emerge:
- Pressure increases with depth: As one descends deeper into a fluid body like water, there is an increase in pressure exerted from above due to the weight of overlying layers.
- Archimedes’ principle: Objects immersed in a fluid experience an upward buoyant force equal to the weight of displaced fluid. If this buoyant force exceeds or equals the object’s weight, it will float; otherwise, it sinks.
- Fluid flow around objects: When an object moves through a fluid medium such as air or water, its shape and speed affect how surrounding fluid flows around it. Understanding this flow pattern enables engineers to design streamlined shapes that minimize drag and optimize efficiency.
- Bernoulli’s principle: Describing how pressure changes with varying speeds of flowing fluids, Bernoulli’s principle explains why airplanes can fly and ships can sail faster when certain conditions are met.
Utilizing these principles allows scientists and engineers to design structures that float or glide through fluids with optimal efficiency and stability. By understanding the interplay of pressure, buoyancy, and fluid flow, we gain insights into various phenomena observed in floating objects and can further explore the concept of fluid flow.
Transitioning seamlessly into our next section on “Exploring the concept of fluid flow,” we will delve deeper into how different factors influence the behavior of fluids as they move around obstacles or interact within confined spaces. This exploration will shed light on intricate dynamics that shape not only floating but also a myriad of other fluid-related phenomena.
Exploring the concept of fluid flow
Transitioning from the previous section on the role of pressure in fluid motion, we now delve into exploring the concept of fluid flow. To illustrate this, let us consider a hypothetical scenario: imagine a small boat gently floating on the surface of a calm lake, undisturbed by any external forces. As we observe this tranquil scene, it becomes apparent that there is more to understand about how fluids interact with objects within them.
Fluid mechanics encompasses various principles and phenomena that govern the behavior of liquids and gases. When it comes to floating objects, several factors contribute to their stability and movement through the fluid medium. Understanding these dynamics is crucial for applications ranging from naval architecture to marine ecology.
To gain insight into fluid flow around floating bodies, we must take into account some key aspects:
- Buoyancy: The upward force exerted on an object immersed in a fluid depends on its volume and density relative to that of the surrounding medium.
- Drag: The resistance encountered by an object moving through a fluid is influenced by its shape, size, and velocity. Factors such as viscosity can significantly impact drag forces.
- Surface interactions: The properties of both the object’s surface and the surrounding fluid influence how they interact. For example, rough surfaces tend to create more turbulence compared to smooth ones.
- Boundary layer effects: At the interface between an object and a fluid, a thin layer known as the boundary layer forms. This region plays a crucial role in determining frictional forces acting on the object.
In order to further comprehend these concepts related to fluid flow around floating objects, let us examine Table 1 below:
Table 1:
Factor | Influence | Example |
---|---|---|
Object shape | Determines drag forces experienced by the body | A streamlined hull minimizes drag while maintaining stability |
Fluid velocity | Affects both buoyancy and drag forces | Increased water currents can alter the floating position |
Surface roughness | Influences the creation of turbulence and boundary layer effects | Barnacles on a boat’s hull increase drag and fuel consumption |
Fluid viscosity | Determines how easily the fluid flows around objects | High-viscosity liquids, like molasses, create more resistance |
In light of these considerations, it becomes evident that understanding fluid flow dynamics is essential for comprehending the behavior of floating objects. In our subsequent section, we will explore the relationship between velocity and pressure in fluids as we continue to unravel the fascinating world of fluid mechanics.
[The next section: The Relationship Between Velocity and Pressure in Fluids]
The relationship between velocity and pressure in fluids
The Dynamics of Fluid Mechanics in Floating: The Relationship between Velocity and Pressure
Continuing from our exploration of fluid flow, let us now delve into the intriguing relationship between velocity and pressure in fluids. To illustrate this concept, imagine a scenario where a small boat is floating peacefully on the surface of a lake. As the wind picks up speed, it generates an airflow that passes over the sail of the boat. This airflow exerts a force on the sail, causing it to move forward.
Understanding this phenomenon requires an understanding of Bernoulli’s principle, which states that as the velocity of a fluid increases, its pressure decreases (and vice versa). In our example with the boat and sail, as air flows faster over one side of the sail compared to the other, there is a difference in velocities between these two regions. According to Bernoulli’s principle, this difference creates a pressure gradient across the sail. Consequently, higher pressure pushes against lower pressure, propelling the boat forward.
To further grasp how velocity and pressure interact in fluids, consider these key points:
- As fluid accelerates through a constriction such as a narrow pipe or nozzle, its velocity increases while its pressure decreases.
- Conversely, when fluid decelerates due to expansion or widening of its pathway, its velocity decreases while its pressure increases.
- The shape and geometry of objects submerged in fluid can also affect their interaction with velocity and pressure gradients.
- Understanding these principles is crucial for engineers designing streamlined structures like submarines or aircraft wings to minimize drag forces.
Let us visualize these concepts using a table highlighting different scenarios:
Scenario | Velocity Change | Pressure Change |
---|---|---|
Narrowing of pathway | Increase | Decrease |
Widening of pathway | Decrease | Increase |
Flow around obstacles | Variable | Variable |
Streamlined structures | Controlled change | Optimized performance |
By comprehending the intricate relationship between velocity and pressure in fluids, scientists and engineers can develop innovative solutions to improve efficiency, optimize designs, and enhance fluid dynamics. In the subsequent section about “The effect of fluid properties on floating objects,” we will explore how different fluid characteristics impact the behavior of objects floating within them.
The effect of fluid properties on floating objects
The Relationship Between Velocity and Pressure in Fluids
In the previous section, we explored the intricate connection between velocity and pressure in fluids. Now, let us delve into another fascinating aspect of fluid mechanics as it relates to floating objects: the effect of fluid properties.
Imagine a scenario where you have two identical boats floating on different bodies of water – one on calm lake waters, and the other on turbulent ocean waves. Despite their identical structures, these boats will experience contrasting forces due to the varying properties of the fluids they are immersed in.
To better understand this phenomenon, consider the following factors that influence how fluid properties affect floating objects:
-
Density: The density of a fluid plays a crucial role in determining buoyancy. A denser fluid exerts greater upward force on an object, making it more likely to float. Conversely, a less dense fluid will provide less support, potentially causing the object to sink.
-
Viscosity: Viscosity refers to a fluid’s resistance to flow. In highly viscous fluids such as honey or molasses, objects may encounter increased drag forces that hinder their ability to float smoothly. On the other hand, low-viscosity fluids like water allow for easier movement through reduced frictional effects.
-
Surface Tension: The cohesive forces present at the surface of a liquid form what is known as surface tension. This property can significantly impact the stability of floating objects by either increasing or decreasing their tendency to remain afloat.
-
Temperature: Changes in temperature can alter not only the viscosity but also the density of a fluid. For instance, heating up air within an inflatable raft decreases its density relative to colder outside air, resulting in increased buoyant force and improved flotation capabilities.
Now let’s take a closer look at these various factors influencing floating objects’ behavior through an emotional lens:
Factors | Emotional Response |
---|---|
Density | Stability |
Viscosity | Resistance |
Surface Tension | Cohesion |
Temperature | Adaptability |
As we consider the emotional responses evoked by these factors, it becomes apparent that fluid properties have a profound impact on how floating objects interact with their environment. Understanding this interplay is crucial for engineers and scientists seeking to design more efficient and reliable watercraft.
In the subsequent section, we will analyze the forces acting on floating bodies, drawing upon our understanding of fluid mechanics to further explore this captivating topic. By examining these forces, we can gain deeper insights into the complex dynamics at play in various flotation scenarios.
Analyzing the forces acting on floating bodies
Transitioning from our previous discussion on the effect of fluid properties on floating objects, we now turn our attention to analyzing the impact of fluid viscosity on the dynamics of floating bodies. To illustrate this concept, let us consider a hypothetical scenario involving two identical wooden blocks placed in different fluids with varying viscosities.
In the first case, Block A is immersed in a highly viscous liquid while Block B experiences buoyancy in a less viscous medium. The contrasting behavior observed between these two scenarios highlights the significance of fluid viscosity in determining the floating characteristics and subsequent motion of objects.
Understanding how fluid viscosity influences floating dynamics is essential for various applications across industries. Here are some key factors that contribute to this phenomenon:
- Resistance forces: A highly viscous fluid exerts greater resistance against an object’s movement through it compared to a low-viscosity one. This increased resistance can significantly alter the path and speed at which an object floats or drifts.
- Drag coefficient: Different fluids possess distinct drag coefficients, indicating how effectively they impede an object’s motion. Higher viscosity fluids generally exhibit higher drag coefficients, resulting in altered trajectories and reduced velocities.
- Stability considerations: The viscosity of the surrounding fluid affects stability by influencing both static equilibrium (when an object remains stationary) and dynamic equilibrium (when an object oscillates). Understanding how changing viscosities influence stability is crucial for designing stable structures and ensuring their safety.
- Hydrodynamic interactions: When multiple objects float within close proximity, high-fluid viscosity can lead to more pronounced hydrodynamic interactions between them. These interactions generate complex flow patterns that affect each body’s behavior differently.
To further comprehend the relationship between fluid viscosity and floating dynamics, Table 1 provides a comparative overview:
Table 1: Effects of Fluid Viscosity on Floating Dynamics
High-Viscosity Fluids | Low-Viscosity Fluids | |
---|---|---|
Drag Coefficient | Higher drag coefficients | Lower drag coefficients |
Object Trajectory | Altered trajectory and reduced velocities | Less influence on object’s path and speed |
Stability | Enhanced stability due to increased resistance | Potential decrease in stability |
Hydrodynamic | Pronounced hydrodynamic interactions | Minimal impact on inter-object interactions |
Understanding the implications of fluid viscosity is crucial for predicting and manipulating floating dynamics effectively. By recognizing the role it plays, engineers and researchers can develop innovative designs that optimize performance while accounting for variations in surrounding fluids.
Transitioning into the subsequent section, we now delve into another critical factor impacting floating dynamics: surface tension.
The impact of surface tension on floating dynamics
Transitioning from our previous discussion on analyzing the forces acting on floating bodies, we now turn our attention to another crucial factor influencing their dynamics – surface tension. To illustrate its significance, let us consider an intriguing example involving a small paper clip placed carefully onto the surface of water.
Surface tension, as observed in this scenario, arises due to cohesive forces between liquid molecules at the air-liquid interface. When the paper clip is gently positioned, it rests atop the water without sinking or immediately dispersing. This remarkable phenomenon can be attributed to surface tension’s ability to create a thin elastic film across the water’s surface, effectively supporting and confining objects that have lower density than that of the liquid itself.
To comprehend how surface tension affects floating dynamics more comprehensively, let us delve into its key characteristics:
- Cohesion: Surface tension is a result of intermolecular attractions within the liquid phase. These strong cohesive forces cause liquid molecules at the surface to exert an inward pull on neighboring molecules, creating a “skin” with higher energy compared to deeper regions.
- Capillary Action: Surface tension allows liquids to defy gravity by climbing up narrow tubes against gravitational force through capillary action—a process where adhesive forces between liquid and solid surfaces overcome gravitational resistance.
- Meniscus Formation: In curved containers such as test tubes or graduated cylinders, surface tension causes menisci—the upward or downward curvature of a liquid’s free surface—due to differences in cohesive interactions along different surfaces.
- Breakage Threshold: Exceeding the limits of surface tension can lead to rupture or breakage when external factors like pressure or disruptive forces overwhelm the attractive forces holding the liquid together.
Taking these aspects into account enables us to appreciate how nuanced and intricate floating dynamics become when influenced by surface tension’s impact. Understanding this behavior grants researchers and engineers valuable insights for designing efficient flotation devices and improving various applications in fields such as naval architecture, oil spill mitigation, and material science.
Transitioning seamlessly into the subsequent section examining the role of viscosity in fluid motion, we continue our exploration of fluid mechanics to unravel further complexities underlying floating phenomena.
Examining the role of viscosity in fluid motion
In our exploration of fluid mechanics, we now turn to examining the role of viscosity in fluid motion. To illustrate its significance, let us consider a hypothetical scenario where an oil spill occurs in the ocean. The spilled oil forms a slick on the water’s surface, spreading out and creating a thin layer that appears colorful under sunlight. This example showcases how viscosity influences the behavior of fluids, particularly when it comes to their ability to flow.
To better understand the impact of viscosity on fluid motion, several key factors come into play:
-
Viscosity contrast: When two fluids with varying viscosities interact, such as motor oil and water mixing during an accidental spillage, distinct behaviors can be observed due to their different resistance to flow. Understanding this interaction is essential for predicting how substances will disperse or separate within a given system.
-
Flow rate determination: Viscosity plays a crucial role in governing the velocity at which fluids move through channels or pipes. For instance, thickening agents added to paints help control their consistency and ensure easy application by adjusting their viscosity. By studying these phenomena, engineers can create efficient systems for various applications like pipelines or transportation networks.
-
Energy dissipation: Fluids with higher viscosity tend to dissipate more energy when flowing compared to less viscous ones. This phenomenon has implications across multiple fields; for instance, understanding energy dissipation helps optimize propeller design in marine engineering or improve fuel efficiency in automotive industries.
-
Biological implications: Viscosity also affects biological processes occurring within organisms’ bodies. From blood circulations and digestion to joint lubrication, understanding how different fluids react inside living systems aids medical professionals in diagnosing diseases and developing treatments.
To further emphasize the importance of viscosity dynamics, consider Table 1 below:
Scenario | Low Viscosity Fluid | High Viscosity Fluid |
---|---|---|
Flow Behavior | Rapid, turbulent flow with less resistance to motion | Slow, laminar flow with higher resistance to motion |
Energy Dissipation | Low energy dissipation due to lesser internal friction | High energy dissipation due to increased internal friction |
Applications | Efficient for transportation and mixing processes | Effective in lubrication and damping mechanisms |
Table 1: A comparison of fluid behaviors based on viscosity.
In summary, understanding the role of viscosity is crucial for comprehending how fluids interact and move. From determining flow rates in industrial applications to exploring biological systems’ mechanics, viscosity dynamics have a profound impact on numerous fields.
[Transition Sentence]: Turning our attention now towards unravelling the phenomenon of capillary action…
Unraveling the phenomenon of capillary action
Examining the role of viscosity in fluid motion has provided valuable insights into understanding various phenomena related to floating. Now, let us delve deeper into the intriguing phenomenon of capillary action and its implications in fluid mechanics.
Consider a hypothetical scenario where a small glass tube is dipped into a container filled with water. As we observe closely, we notice that the liquid rises inside the tube against gravity, defying our intuition. This mesmerizing process can be attributed to capillary action, which occurs due to cohesive forces between molecules and adhesive forces between the liquid and solid surfaces.
To comprehend this phenomenon better, it is essential to explore some key aspects associated with capillary action:
-
Capillary rise: The height to which a liquid ascends within a narrow tube depends on factors such as surface tension, contact angle, and radius of the tube. These interdependent variables contribute collectively to determine how high or low the liquid will rise within the capillary.
-
Meniscus formation: When a liquid forms an interface with another substance (such as air or solid), it develops what is known as a meniscus. The shape of this meniscus plays a crucial role in determining both the extent of capillary rise and other characteristics specific to each type of liquid.
-
Impact on porous materials: Capillary action influences how fluids interact with porous substances like sponges, fabrics, or paper towels. By exploiting these properties effectively, absorption-based technologies have been developed for applications ranging from medical devices to environmental remediation processes.
-
Wide-ranging applications: Understanding capillarity aids in comprehending diverse natural phenomena and engineering applications alike. From trees drawing water through their roots via capillaries in xylem tissues to ink pens relying on capillary flow mechanisms for smooth writing experiences—capillary action finds relevance across multiple domains.
The table below highlights some examples showcasing real-world scenarios where capillarity plays a significant role:
Application | Description | Implications |
---|---|---|
Soil moisture | Capillary action helps plants access water | Essential for plant growth |
Blood circulation | Capillaries facilitate nutrient exchange | Vital for overall body functioning |
Paper absorption | Porous nature of paper aids capillary rise | Enables efficient ink or liquid uptake |
Fuel cell systems | Capillary forces enhance reactant transport | Improves performance and efficiency |
As we have seen, capillary action is a fascinating phenomenon that holds immense relevance in various fields. In the subsequent section, we will explore the influence of Stokes law on floating objects, shedding light on yet another intriguing aspect of fluid mechanics.
[Transition Sentence] Moving forward, let us now examine the influence of Stokes law on floating objects, which further expands our understanding of fluid dynamics and its implications.
The influence of Stokes law on floating objects
The Influence of Stokes Law on Floating Objects
Having examined the phenomenon of capillary action in detail, we now turn our attention to another important aspect of fluid mechanics in floating: the influence of Stokes law. To illustrate this concept, let us consider a hypothetical scenario involving a small wooden block placed at the surface of water.
As the wooden block is released into the water, it starts to sink due to its density being greater than that of water. However, as it descends further into the liquid, an interesting phenomenon occurs. The drag force acting on the submerged part of the block increases proportionally with its velocity and size, while buoyancy provides an opposing upward force. Eventually, these forces reach equilibrium, causing the object to float at a certain depth.
To gain a deeper understanding of how Stokes Law influences floating objects, we can explore several key factors:
- Size and shape: Larger objects experience higher drag forces due to their increased surface area exposed to fluid flow.
- Velocity: Faster-moving objects generate stronger drag forces which may hinder their ability to float.
- Density difference: If an object’s density closely matches that of the fluid it occupies, minimal displacement occurs and flotation becomes more likely.
- Viscosity: Fluids with high viscosity impose greater resistance on moving objects, affecting their ability to remain buoyant.
In considering these factors collectively, we can visualize their interplay by referring to the following table:
Factors | Effect on Floating |
---|---|
Size/Shape | Increased Drag |
Velocity | Greater Resistance |
Density Difference | Enhanced Buoyancy |
Viscosity | Impeded Flotation |
Understanding how these factors interact allows us to comprehend why some objects effortlessly float while others sink. It also underscores the delicate balance between various forces involved in maintaining an object’s position within a fluid medium.
In preparation for exploring practical applications related to Bernoulli’s principle in floating, we now shift our focus to the next section. Here, we will delve into how this fundamental principle influences the behavior of objects at the interface between air and water.
[Transition sentence into the subsequent section about “The practical applications of Bernoulli’s principle in floating”] By examining these applications, we can further appreciate the intricate relationship between fluid mechanics and the dynamics of floating objects.
The practical applications of Bernoulli’s principle in floating
Transitioning smoothly from the previous section exploring the influence of Stokes law on floating objects, we now delve into another fundamental concept in fluid mechanics that finds practical applications in floating – Bernoulli’s principle. By understanding how this principle operates, we can gain insights into various phenomena related to buoyancy and stability.
To illustrate the practical relevance of Bernoulli’s principle, consider a scenario where an airship is hovering above the ground. The shape of the airship plays a crucial role in its ability to stay airborne. The design incorporates principles based on Bernoulli’s theorem to create different pressure zones around it. For instance:
- As the air flows over the curved upper surface of the airship, it travels faster and creates lower pressure compared to the stationary lower surface.
- This pressure difference generates lift force, allowing the airship to remain buoyant against gravity.
Applying Bernoulli’s principle extends beyond just airships; numerous other examples demonstrate its significance in floating mechanisms. Some notable applications include:
- Sailboats: By utilizing differences in wind speed between both sides of a sail, sailors adjust their sails accordingly to generate lift force and propel their boats forward.
- Submarines: To control depth underwater, submarines employ ballast tanks that manipulate water levels inside them using Bernoulli’s principle.
- Hydrofoils: These boat attachments use wings or foils underneath their hulls, altering water flow patterns through varying speeds to reduce drag and enhance stability.
- Hovercrafts: Employing high-pressure fans beneath them, hovercrafts create cushions of air that adhere to Bernoulli’s principle for achieving buoyancy while gliding smoothly over land or water.
These real-world applications vividly showcase how engineers harness Bernoulli’s principle as a valuable tool when designing floating structures.
Application | Description | Principle Used |
---|---|---|
Airships | Employing pressure differences between upper and lower surfaces to generate lift force. | Bernoulli’s theorem |
Sailboats | Utilizing wind speed variations across sails for generating forward propulsion. | Bernoulli’s principle |
Submarines | Controlling depth underwater by manipulating water levels inside ballast tanks through pressure differentials. | Bernoulli’s equation |
Hydrofoils | Reducing drag and enhancing stability through wings or foils that alter water flow patterns using Bernoulli’s principles. | Fluid dynamics |
By examining the practical applications of Bernoulli’s principle in floating, we gain a deeper understanding of how fluid mechanics aids in achieving buoyancy and stability in various objects and vessels.
In summary, this section explored the practical utilization of Bernoulli’s principle in floating mechanisms such as airships, sailboats, submarines, and hydrofoils. These examples vividly demonstrate the ways engineers employ this fundamental concept from fluid mechanics to design structures capable of staying afloat while maintaining stability. Understanding these applications allows us to appreciate the intricate relationship between fluid dynamics and floating systems without compromising their efficiency or effectiveness.