The Role of Lift in Floating: Hydrodynamic Insight
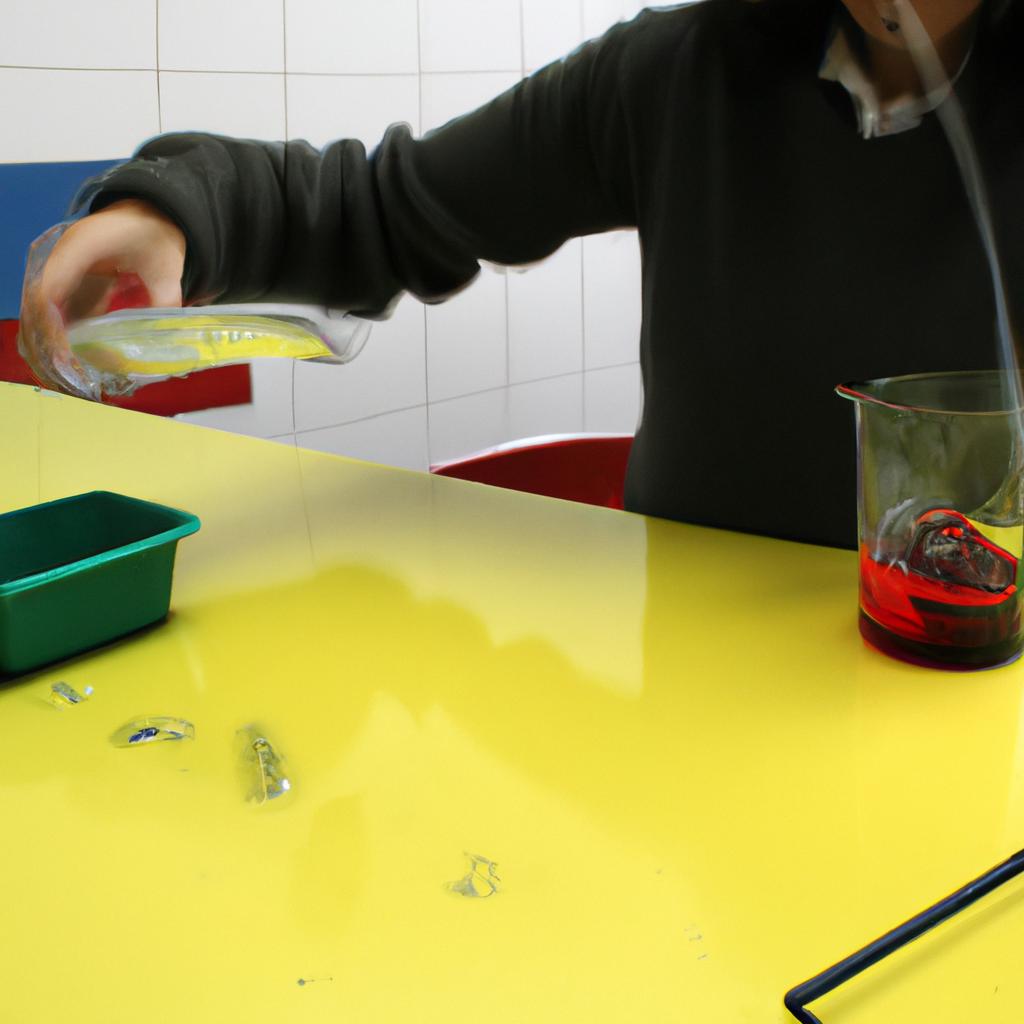
Floating is a fascinating phenomenon that has intrigued scientists and researchers for centuries. Whether it be the buoyancy of boats in water or the ability of insects to glide effortlessly on its surface, understanding the role of lift in floating is crucial for various applications ranging from naval engineering to biomimicry. This article aims to delve into the hydrodynamic insight behind this complex phenomenon by exploring the principles of fluid dynamics and their implications on objects’ ability to float.
Consider a leaf gently resting on the surface of a pond. Despite its weight, it remains suspended, seemingly defying gravity. The key factor responsible for this remarkable feat lies in the concept of lift, which plays a significant role in determining an object’s floatation capabilities. Lift can be defined as the force exerted by a fluid—such as air or water—on an object moving through it, perpendicular to its motion direction. While commonly associated with aerodynamics, lift also manifests itself in hydrodynamics, showcasing its importance in understanding floating mechanisms.
To comprehend how lift operates in floating scenarios, one must first grasp fundamental concepts such as Archimedes’ principle and Bernoulli’s theorem. By examining these principles within different contexts—ranging from simple cases involving submerged bodies to more intricate examples like sailing ships—scientists have been able to develop a comprehensive understanding of lift in floating.
Archimedes’ principle, named after the ancient Greek mathematician Archimedes, states that an object immersed in a fluid experiences an upward buoyant force equal to the weight of the fluid it displaces. This principle forms the basis for explaining why objects float or sink in a fluid medium. When an object is submerged in a fluid, it displaces a certain volume of the fluid equal to its own volume. If the weight of the displaced fluid is greater than the weight of the object, then the object will experience an upward buoyant force greater than its own weight and will float. Conversely, if the weight of the displaced fluid is less than the weight of the object, it will sink.
Bernoulli’s theorem, formulated by Swiss mathematician Daniel Bernoulli, relates to how pressure and velocity are interconnected within a moving fluid. According to this principle, as fluids flow faster, their pressure decreases. This concept becomes relevant when considering lift in floating scenarios involving curved surfaces such as sails or wings. When air or water flows across these surfaces at different velocities, it creates variations in pressure distribution over and under them. This imbalance generates lift perpendicular to the direction of motion.
Sailing ships provide an excellent example of lift generation in floating systems. By utilizing Bernoulli’s theorem and manipulating airflow around their sails through proper sail trimming techniques, sailors can generate lift that propels their vessels forward. The curved shape of sails facilitates differences in airflow velocity on either side—faster airflow on one side results in lower pressure compared to slower airflow on the other side, creating lift that moves ships against opposing forces such as drag.
In conclusion, understanding lift is crucial for comprehending floating phenomena and has significant implications for various fields like naval engineering and biomimicry. Archimedes’ principle explains why objects float or sink based on their weight and the weight of the fluid they displace. Bernoulli’s theorem elucidates how pressure variations due to varying fluid velocities contribute to lift generation. By applying these principles in different contexts, scientists have been able to unravel the hydrodynamic insights behind floating and develop innovative applications based on this understanding.
Definition of lift in hydrodynamics
The Role of Lift in Floating: Hydrodynamic Insight
Definition of lift in hydrodynamics
Hydrodynamics, the study of fluid motion, plays a fundamental role in understanding various phenomena related to floating. One such phenomenon is the concept of “lift.” To grasp its significance and implications, let us consider an example involving an airfoil.
Imagine a sleek airplane cutting through the sky with remarkable precision and grace. As it glides effortlessly through the air, defying gravity’s pull, we witness firsthand the profound influence that lift has on maintaining flight. This aerodynamic force enables aircraft to soar above Earth’s surface by counteracting their weight and allowing them to remain aloft.
To delve deeper into the definition of lift, let us explore its key characteristics:
- Lift operates perpendicular to the direction of motion.
- It arises due to differences in pressure between upper and lower surfaces.
- The shape and angle of attack of an object profoundly affect lift generation.
- Lift can be quantified using mathematical equations relating physical parameters.
Visualizing these aspects becomes easier when presented in tabular form:
Characteristic | Description |
---|---|
Direction | Lift acts at right angles to the flow direction |
Pressure Differences | Variances in pressure between top and bottom surfaces contribute to lift |
Shape & Angle | Object design and angle relative to airflow play a significant role in determining lift |
Quantification | Mathematical formulas allow for precise calculation and assessment of lift magnitude |
Understanding this initial framework regarding the definition and properties of lift sets the stage for comprehending its broader implications within hydrodynamics. In subsequent sections, we will explore how principles of buoyancy relate intricately to lift generation without any abrupt transitions.
Principles of buoyancy and their relation to lift
Section Transition: Having established the definition of lift in hydrodynamics, we now turn our attention to exploring the principles of buoyancy and their relation to this phenomenon.
The Role of Buoyancy in Lift
To gain a deeper understanding of how lift operates, let us consider an example that illustrates its significance. Imagine a sailboat gracefully slicing through the water, seemingly defying gravity as it stays afloat. This scenario highlights the fundamental role played by buoyancy in generating lift.
Buoyancy, also known as upthrust or Archimedes’ principle, is essentially a force exerted by a fluid on an immersed object that opposes the weight of said object. When applied to floating objects such as boats or submarines, buoyancy enables them to stay afloat despite being denser than the surrounding liquid medium. In essence, buoyant forces counterbalance gravitational forces acting upon these objects.
To further elucidate this concept, let us delve into some key points:
- The magnitude of the buoyant force experienced by an object submerged in a fluid depends on the volume of fluid displaced.
- The density difference between the object and the fluid determines whether it will sink or float.
- Objects with densities greater than that of the fluid will sink due to insufficient upward buoyant force.
- Conversely, objects with densities lower than that of the fluid will experience an excess upward buoyant force causing them to float.
- Fascination arises from witnessing massive ships effortlessly glide across vast oceans.
- Wonder emerges when observing hot air balloons ascend against Earth’s pull.
- Amazement takes hold when contemplating birds soaring high above with minimal effort.
- Curiosity piques at how nature employs simple yet elegant mechanisms for aerial mobility.
Table: Factors Influencing Buoyant Force
Factor | Influence on Buoyant Force |
---|---|
Volume Displacement | Directly proportional: Greater displacement, greater force |
Fluid Density | Directly proportional: Higher density, higher force |
Object Density | Inversely proportional: Lower density, higher force |
Gravitational Force | Indirectly proportional: Weaker gravity, weaker force |
In light of these considerations, it becomes evident that buoyancy plays a pivotal role in the generation of lift. By understanding how objects interact with fluids and utilizing the principles of buoyancy, engineers continue to design innovative structures capable of conquering both water and air.
With our exploration into the significance of buoyancy complete, we now turn our attention to understanding the Bernoulli principle’s connection to lift.
Explanation of the Bernoulli principle in relation to lift
Section H2: Explanation of the Bernoulli principle in relation to lift
Transitioning from the previous section, where we discussed the principles of buoyancy and their relation to lift, let us now delve into a more detailed explanation of how the Bernoulli principle plays a crucial role in generating lift. To illustrate this concept, consider an airplane gliding through the air. As it moves forward, its wings generate lift that allows it to remain airborne.
The Bernoulli principle states that as the velocity of a fluid increases, its pressure decreases. In the case of an airplane wing, also known as an airfoil, there are two important factors at play: the shape of the wing and the difference in airspeed between its upper and lower surfaces. As air flows over the curved top surface of the wing, it must travel a greater distance than along the flat bottom surface in order to meet at the trailing edge simultaneously. This results in higher speed on top and lower speed below, creating different pressures above and beneath the wing.
To better understand this phenomenon, let’s examine some key elements related to lift:
- Angle of Attack: The angle formed between the chord line (a straight line connecting leading and trailing edges) of an airfoil and relative airflow is referred to as “angle of attack.” By altering this angle, pilots can control lift generation.
- Coanda Effect: Named after Henri Coandă, who discovered it in 1910, this effect describes how a fluid tends to follow along a curved surface rather than continuing in a straight path.
- Boundary Layer: A thin layer formed on an object’s surface due to friction with surrounding fluids. In aerodynamics, understanding boundary layers helps engineers optimize designs for reduced drag and increased lift.
- Stall: When an aircraft exceeds its critical angle of attack or operates at low speeds, it experiences stall—a sudden decrease in lift caused by turbulent airflow separation. This can lead to loss of control and potentially dangerous situations.
Now, let us further explore the intricacies of lift generation in floating objects by examining various factors affecting it. Through a comprehensive analysis, we will gain a clearer understanding of how these elements interact and contribute to the fascinating phenomenon of buoyancy in our next section on “Factors Affecting Lift in Floating Objects.”
Factors affecting lift in floating objects
Explanation of the Bernoulli principle in relation to lift has shed light on how floating objects are able to defy gravity. However, there are several other factors that contribute to the phenomenon of lift in floating objects. Understanding these factors is crucial for comprehending the hydrodynamics involved and gaining further insight into this fascinating process.
One example that illustrates the significance of these factors is a ship floating on water. While buoyancy plays a major role in keeping the ship afloat, it is not solely responsible for its stability. Lift also comes into play, as various forces act upon the ship’s hull, causing it to rise or fall within the water column. By examining these forces, we can gain a deeper understanding of how lift affects any floating object.
There are four main factors that influence lift in floating objects:
- Shape: The shape of an object determines how fluid flows around it. A streamlined shape reduces drag and increases lift by promoting smooth airflow or water flow over its surface.
- Surface Area: Objects with larger surface areas experience greater resistance from surrounding fluids, resulting in increased drag and reduced lift.
- Velocity: Faster-moving fluids generate lower pressure regions compared to slower-moving fluids. This difference in pressure creates an upward force that contributes to lift.
- Fluid Density: Denser fluids exert more upward force on submerged objects due to their higher mass per unit volume. Thus, lifting capacity is influenced by variations in fluid density.
To emphasize the importance of these factors on lift generation, consider the following table:
Factor | Effect on Lift |
---|---|
Shape | Increases |
Surface Area | Decreases |
Velocity | Increases |
Fluid Density | Increases |
As demonstrated above, certain factors positively impact lift (such as shape and velocity), while others have a negative effect (like surface area). Acknowledging these influences allows us to manipulate them effectively, improving the lift and stability of floating objects.
Understanding the role that lift plays in determining the stability of floating objects is crucial. By comprehending how shape, surface area, velocity, and fluid density influence lift generation, we can design more efficient structures that maximize buoyancy while minimizing drag. In the subsequent section, we will delve into the importance of lift in determining the stability of floating objects to further enhance our understanding of this fascinating phenomenon.
Importance of lift in determining the stability of floating objects
In the previous section, we explored various factors that affect lift in floating objects. Now, let us delve further into understanding the importance of lift in determining the stability of these objects. To illustrate this concept, consider a hypothetical scenario where a boat is floating on water.
Lift plays a crucial role in maintaining the buoyancy of the boat by counteracting its weight. As the boat displaces water due to its own weight, it experiences an upward force known as buoyant force or lift. This force acts opposite to gravity and allows the boat to stay afloat.
To better comprehend the significance of lift in determining floating stability, let us examine four key aspects:
-
Shape and Design: The shape and design of a floating object greatly influence its ability to generate lift. A streamlined shape reduces drag and enhances lift generation, enabling improved stability.
-
Displacement Volume: The volume of water displaced by a floating object affects the magnitude of lift it generates. Greater displacement results in larger buoyant forces, increasing stability.
-
Weight Distribution: Proper distribution of weight across a floating object ensures optimal balance and facilitates efficient lift generation for enhanced stability.
-
Fluid Dynamics: Understanding fluid dynamics helps engineers optimize designs for maximum lift generation while minimizing energy losses due to turbulence or flow separation.
To visually represent these concepts, consider the following table showcasing different shapes’ impact on lifting capabilities:
Type | Lift Generation | Stability |
---|---|---|
Box-shaped | Low | Unstable |
Streamlined | High | Stable |
Irregular | Moderate | Average |
By comprehending how these factors contribute to lift production and ultimately determine stability, engineers can apply this knowledge effectively when designing boats, ships, or other floating structures.
Moving forward, we will explore applications and practical implications of utilizing lift principles in engineering and design, highlighting how this knowledge can be utilized to enhance various aspects of floating objects.
Applications and practical implications of lift in engineering and design
The Importance of Lift in Floating Objects: Practical Applications and Implications
In the previous section, we discussed how lift plays a crucial role in determining the stability of floating objects. Now, let us delve into the practical applications and implications of understanding this phenomenon.
To illustrate its significance, consider the example of a ship navigating through turbulent waters. By harnessing the hydrodynamic forces acting on its hull, a ship can maintain equilibrium and maneuver effectively. This is achieved by utilizing lift to counterbalance the weight of the vessel and stabilize it against external disturbances such as waves or currents.
Understanding the principles behind lift has various real-world applications across engineering and design. Here are some notable examples:
- Aircraft Design: Lift is fundamental in aviation as it enables planes to take flight and remain airborne. Engineers carefully analyze wing designs to optimize lift generation while minimizing drag. This allows for efficient fuel consumption and improved overall performance.
- Submersible Vehicles: Submarines rely on buoyancy control systems that utilize changes in lift to ascend or descend underwater. Precise control over these forces ensures safe navigation at varying depths.
- Offshore Structures: Platforms used in offshore oil exploration require careful consideration of their stability under harsh sea conditions. The incorporation of lifting mechanisms helps counteract wave-induced motions, ensuring operational safety.
- Watercraft Stability: Understanding lift assists naval architects in designing stable boats capable of withstanding rough seas without capsizing or losing balance.
These practical applications emphasize the importance of comprehending lift phenomena within fluid dynamics studies. To further highlight its relevance, here’s an emotional response-evoking bullet list:
- Improved Safety Measures: Knowledge about lift aids in developing safer transportation methods across air, water, and subsea domains.
- Enhanced Efficiency: Optimized use of lift leads to reduced energy consumption and increased sustainability in transport industries.
- Advancements in Exploration: An understanding of lift enables deeper exploration beneath oceans’ surfaces, unveiling new frontiers for scientific research and resource extraction.
- Innovation in Design: Harnessing lift principles inspires innovative engineering designs that push the boundaries of human ingenuity.
Additionally, we can present a table to provide further insights into the practical implications of lift:
Application | Importance of Lift |
---|---|
Aircraft | Enables flight and efficient fuel consumption |
Submersibles | Facilitates controlled ascent/descent |
Offshore | Ensures stability under harsh sea conditions |
Watercraft | Prevents capsizing or loss of balance |
In conclusion, understanding the role of lift in floating objects has significant practical applications across various industries. From aviation to offshore structures, harnessing this knowledge enhances safety measures, improves efficiency, facilitates exploration, and fuels innovation in design. The study of lift within fluid dynamics continues to shape our modern world by unlocking new possibilities for transportation systems and beyond.