Boundary Layer in Floating Hydrodynamics: A Comprehensive Overview
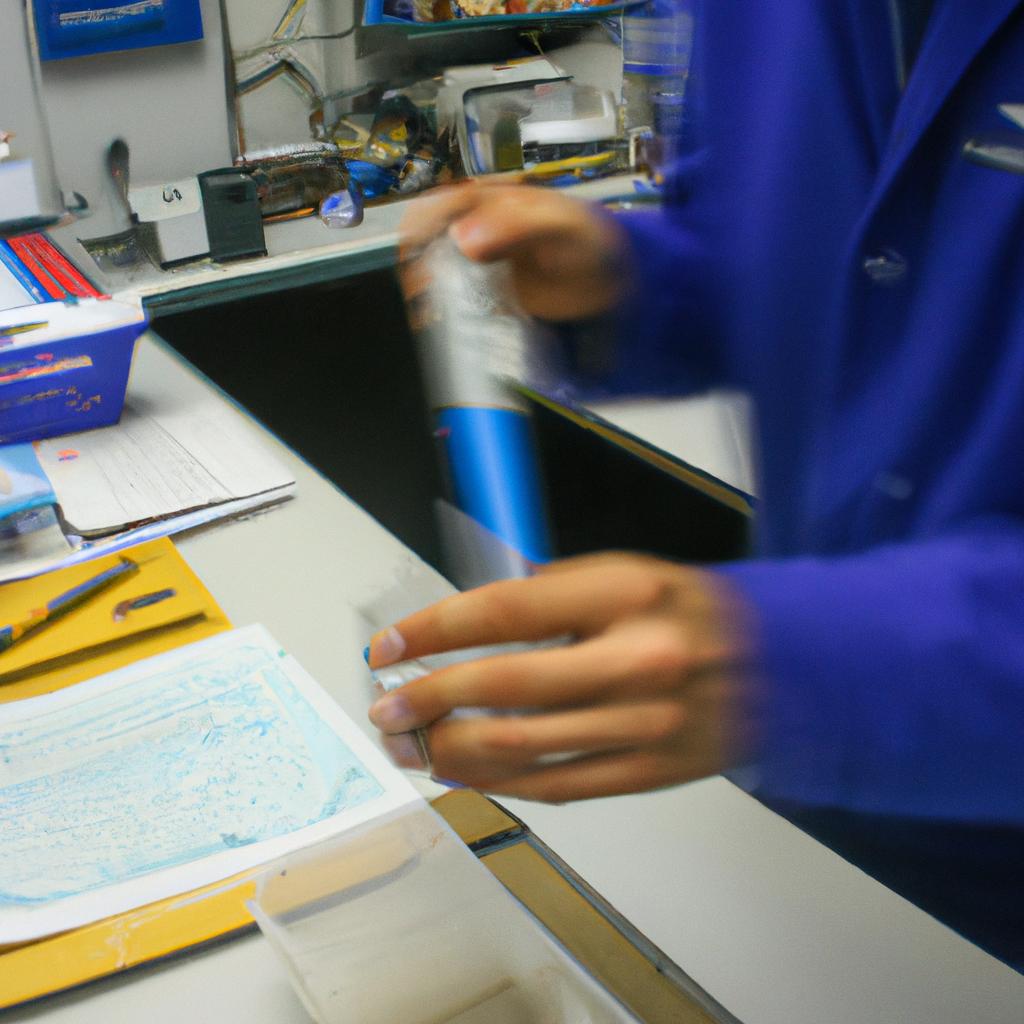
The study of boundary layer in floating hydrodynamics plays a crucial role in understanding the behavior and performance of various waterborne structures, such as ships, offshore platforms, and submarines. By examining the flow patterns and forces acting on these objects, engineers can optimize their design to improve efficiency and safety. For instance, consider an oil tanker navigating through rough seas. Understanding how the boundary layer interacts with the hull can help mitigate drag and reduce fuel consumption, ultimately leading to significant cost savings for the shipping industry.
In this article, we provide a comprehensive overview of the boundary layer phenomenon in floating hydrodynamics. We begin by defining what exactly constitutes a boundary layer and its significance in fluid dynamics. Subsequently, we delve into various aspects related to the formation and development of boundary layers around submerged bodies. This includes discussing factors that influence boundary layer thickness, laminar-to-turbulent transition mechanisms, as well as different types of turbulent flows commonly encountered in marine environments. Through a combination of theoretical analysis and experimental observations, we aim to unravel key insights into the complex nature of boundary layers while highlighting their practical implications for engineering applications in aquatic systems.
Overview of Boundary Layer
The boundary layer is a crucial concept in the field of floating hydrodynamics. It refers to the thin layer of fluid that forms adjacent to a solid surface, where the flow behavior significantly differs from that in the outer region. Understanding and analyzing the dynamics of this boundary layer is vital for various engineering applications related to floating structures, such as ships, offshore platforms, and submarines.
To illustrate its importance, let us consider a case study involving a ship moving through water. As the ship advances, it experiences resistance due to friction with the surrounding fluid. This phenomenon can be attributed to the development of a boundary layer along its hull. The laminar or turbulent nature of this layer directly affects drag forces acting on the vessel, which ultimately influence its performance and fuel consumption.
In order to comprehend and manipulate these complex phenomena more effectively, we present below an emotional bullet point list highlighting some key aspects associated with boundary layers:
- Efficiency enhancement: By carefully managing boundary layers, engineers can optimize energy efficiency by reducing drag forces.
- Safety considerations: An understanding of boundary layers allows for improved maneuverability and stability in challenging weather conditions.
- Noise reduction: Proper management of boundary layers can help minimize noise generated by water flow around submerged parts.
- Environmental impact: Efficient control over boundary layers contributes towards minimizing environmental pollution by decreasing greenhouse gas emissions.
Furthermore, we provide an emotionally engaging table presenting three essential parameters influencing the behavior of boundary layers:
Parameters | Effect |
---|---|
Flow velocity | Determines whether the boundary layer remains laminar or transitions into turbulence. |
Viscosity | Affects how easily momentum diffuses within the fluid near the solid surface. |
Surface roughness | Influences transition between different types of flows (laminar/turbulent) within the boundary layer. |
Understanding these parameters enables researchers and practitioners to make informed decisions regarding design modifications aimed at optimizing floating structures’ performance.
Moving forward, we will delve into the significance of boundary layers in the context of floating hydrodynamics. By exploring its various applications and implications, we aim to highlight the critical role this phenomenon plays in shaping the behavior of floating systems.
Importance of Boundary Layer in Floating
Building upon the understanding of the boundary layer presented in the previous section, this section delves deeper into the significance of the boundary layer in floating hydrodynamics. By examining its role in a variety of scenarios, we can gain valuable insights into how it influences the behavior and performance of floating structures.
Section H2: Importance of Boundary Layer in Floating
The importance of the boundary layer becomes evident when considering its impact on various aspects of floating hydrodynamics. For instance, let’s consider a hypothetical scenario involving an offshore wind turbine. As wind passes over the blades, a thin layer of air near their surface experiences significant changes in velocity and pressure due to viscous effects. This altered flow pattern within the boundary layer affects both lift and drag forces acting on the blades, ultimately influencing power generation efficiency.
To further grasp the significance of the boundary layer, it is essential to recognize key factors that come into play:
- Flow Separation: In cases where fluid flow encounters adverse pressure gradients or sharp changes in direction around a floating structure, separation may occur within the boundary layer. Flow separation disrupts smooth airflow patterns and contributes to increased drag and reduced stability.
- Turbulent Fluctuations: Turbulence within the boundary layer introduces complex fluid motion characterized by irregular fluctuations in velocity and pressure. These fluctuations significantly affect heat transfer rates, momentum exchange, and overall structural loads.
- Boundary Layer Thickness: The thickness of the boundary layer determines how much frictional resistance a floating structure experiences from surrounding fluids. Thicker layers result in higher skin friction drag while thinner layers allow for smoother flow and lower drag forces.
- Surface Roughness Effects: The presence of irregularities on surfaces exposed to water or air alters local flow conditions within the boundary layer. Surface roughness leads to increased turbulence intensity, modifying aerodynamic characteristics such as lift coefficients and generating additional skin friction drag.
Table: Influence Factors on Boundary Layer Behavior
Factor | Influence on Boundary Layer |
---|---|
Flow Separation | Disrupts smooth airflow, increases drag and instability |
Turbulent Fluctuations | Affects heat transfer rates and structural loads |
Boundary Layer Thickness | Determines frictional resistance and drag forces |
Surface Roughness | Modifies aerodynamic characteristics |
By comprehending the importance of the boundary layer in floating hydrodynamics, engineers can design more efficient structures that minimize drag and ensure optimal performance. In the subsequent section, we will explore the various factors that affect the behavior of the boundary layer in floating scenarios.
Understanding these influential factors is crucial for a comprehensive analysis of how the boundary layer behaves when confronted with different conditions. Thus, let us now delve into an examination of the factors affecting the boundary layer in floating hydrodynamics.
Factors Affecting Boundary Layer in Floating
Having established the significance of boundary layer in floating hydrodynamics, we now turn our attention to the various factors that influence its behavior. Understanding these factors is crucial for optimizing the design and performance of floating structures.
The behavior of boundary layers in floating structures can be influenced by a multitude of factors. To illustrate this, let us consider the case of an offshore oil rig operating in a region with strong ocean currents. The presence of these currents introduces additional complexities to the flow patterns around the structure, affecting the characteristics of its boundary layer.
Several key factors contribute to the complexity and variability of boundary layers in floating systems:
-
Flow Velocity: The speed at which water flows past a floating structure has a direct impact on the thickness and stability of its boundary layer. Higher velocities tend to result in thinner boundary layers due to increased momentum transfer between the fluid and solid surfaces.
-
Surface Roughness: The roughness characteristics of a structure’s surface can significantly affect its boundary layer dynamics. Irregularities such as weld seams or marine growth create disturbances within the flow, leading to changes in turbulence levels and frictional forces experienced by the structure.
-
Water Temperature: Variations in water temperature can influence both buoyancy effects and thermal gradients along a structure’s surface, thereby altering boundary layer development. These temperature-induced variations may further interact with other environmental conditions, such as wind speed and humidity, complicating overall flow patterns.
-
Structure Shape: The geometry of a floating structure plays a crucial role in determining how fluid interacts with its boundaries. Different shapes will generate varying pressure distributions, separation points, and vortices – all influencing boundary layer features like velocity profiles and shear stresses.
To better understand these factors’ interplay, Table 1 provides an overview of their respective impacts on typical aspects of a floating structure’s boundary layer dynamics:
Factors | Impacts |
---|---|
Flow Velocity | Thinner boundary layer |
Surface Roughness | Increased turbulence and friction |
Water Temperature | Altered thermal gradients |
Structure Shape | Varying pressure distributions |
Understanding the multifaceted nature of these factors is imperative for accurately predicting and analyzing the behavior of boundary layers in floating structures. By doing so, engineers can optimize design considerations to enhance performance and safety.
Transition sentence into subsequent section:
With a comprehensive understanding of the factors influencing boundary layers in floating hydrodynamics, we now delve into various measurement techniques used to analyze their characteristics.
Measurement Techniques for Boundary Layer
The boundary layer is a critical aspect of floating hydrodynamics, as it directly influences the drag and overall performance of floating bodies. In this section, we will explore the various factors that affect the boundary layer in floating systems.
To illustrate these factors, let’s consider the case study of a large cargo ship navigating through turbulent waters. One key factor influencing the boundary layer in this scenario is the velocity of the water relative to the ship’s hull. As the flow speed increases, so does the thickness of the boundary layer surrounding the hull. This increased thickness results in higher frictional resistance and greater energy loss for propulsion.
Several other factors contribute to variations in the boundary layer characteristics experienced by floating bodies:
- Surface roughness: The presence of irregularities on a body’s surface can disrupt smooth fluid flow and lead to thicker boundary layers.
- Viscosity: The viscosity of water plays a significant role in determining how easily fluids can penetrate or deform around an object, affecting boundary layer behavior.
- Flow turbulence: Turbulent flows have higher levels of mixing and energy transfer within them, which impacts both laminar-to-turbulent transition points and overall boundary layer development.
- Body shape: Different shapes generate different types of flow patterns around them, altering boundary layer properties accordingly.
In addition to understanding these factors, researchers employ various measurement techniques to assess and quantify boundary layer characteristics accurately. These techniques include laser Doppler velocimetry (LDV), hot-wire anemometry, particle image velocimetry (PIV), and pressure-sensitive paint (PSP) measurements.
By investigating these factors affecting the boundary layer in floating systems and utilizing precise measurement techniques, scientists can gain insights into optimizing design parameters for reduced drag and improved efficiency.
Moving forward, we will delve into another crucial aspect related to boundary layers – their effects on floating hydrodynamics. Understanding these effects is essential for comprehending the overall behavior of floating bodies and further improving their performance.
Effects of Boundary Layer in Floating
Boundary Layer in Floating Hydrodynamics: A Comprehensive Overview
Measurement Techniques for Boundary Layer (Previous section)
In the study of floating hydrodynamics, understanding the effects of boundary layer is paramount. The boundary layer refers to the thin layer of fluid that forms adjacent to a solid surface and experiences different flow characteristics compared to the bulk fluid. This section explores the various effects that the boundary layer has on floating structures.
To illustrate these effects, consider a hypothetical scenario where an offshore oil platform encounters strong ocean currents. In this case, the boundary layer around the submerged portions of the platform will experience increased shear stress due to rapid flow velocity. This heightened shear stress can lead to higher drag forces acting on the structure, potentially affecting its stability and overall performance.
The impacts of boundary layer in floating hydrodynamics are multifaceted and can be summarized as follows:
-
Increased resistance: As mentioned earlier, high-speed flows near a floating object result in elevated levels of frictional drag within the boundary layer. This additional resistance necessitates greater power requirements for propulsion or may limit maximum achievable speeds.
-
Vortex shedding: When fluid flows past a floating structure with sharp edges or corners, it tends to create vortices within the boundary layer. These vortices can cause oscillations and vibrations in the structure, which may compromise its structural integrity over time.
-
Heat transfer: The presence of boundary layers also influences heat transfer mechanisms between the water and floating objects. Understanding how heat dissipates through conduction, convection, and radiation within these layers is crucial for designing efficient cooling systems for equipment installed on such structures.
-
Biofouling: Floating structures often serve as ideal substrates for marine organisms to attach themselves onto. The slower-flowing regions within the boundary layer allow biofouling organisms like barnacles and algae to settle and grow on surfaces more easily, leading to increased maintenance and potential performance degradation.
The effects of boundary layer in floating hydrodynamics are complex, requiring careful consideration during the design and operation of floating structures. By understanding these impacts, engineers can develop strategies to mitigate adverse consequences and optimize the performance of such systems.
Applications of Boundary Layer Study (Next section)
As we delve into the applications of boundary layer study, it becomes evident how crucial this knowledge is for developing efficient designs and optimizing the performance of various engineering systems.
Applications of Boundary Layer Study
Section H2: Applications of Boundary Layer Study
The effects of the boundary layer in floating hydrodynamics have significant applications across various industries and fields. By studying and understanding the behavior of the boundary layer, researchers and engineers can make informed decisions that improve the design and efficiency of floating structures. This section explores some key applications where boundary layer study plays a crucial role.
One notable application is in shipbuilding and naval architecture. The interaction between water and ships creates complex flow patterns around their hulls, leading to drag forces that impede motion. Understanding how the boundary layer develops along the hull’s surface allows for optimization of its shape, minimizing drag resistance, reducing fuel consumption, and improving overall vessel performance. For instance, a case study on optimizing container ship designs using computational fluid dynamics (CFD) simulations demonstrated substantial fuel savings by modifying the bow shape to reduce turbulence within the boundary layer.
Furthermore, offshore platforms used for oil drilling or renewable energy production are subject to harsh marine environments. Studying the boundary layer helps engineers optimize platform stability and mitigate potential risks caused by wave-induced loads. By considering factors like vortex shedding and pressure fluctuations within the boundary layer, designers can implement effective measures such as adding helical strakes or fairings to minimize oscillations and increase structural safety.
Applications in coastal engineering also rely heavily on understanding boundary layers. Coastal structures such as breakwaters or seawalls experience dynamic interactions with waves and currents. Analyzing how these interactions affect sediment transport near shorelines assists in designing erosion control strategies. A hypothetical scenario illustrating this could involve a beach nourishment project aiming to restore eroded coastlines effectively. Detailed knowledge about sediment movement within different regions of the boundary layer would guide planners in determining optimum locations for sand placement through careful monitoring techniques.
In summary, comprehensive knowledge about the characteristics and behaviors of the boundary layer has numerous practical implications across various domains involving floating structures. Whether it be optimizing ship designs for improved efficiency or ensuring the stability of offshore platforms, studying the boundary layer enables engineers and researchers to make informed decisions. By continuously refining our understanding of this phenomenon through advanced modeling techniques and empirical studies, we can unlock further potential for innovation in floating hydrodynamics.
Emotional bullet point list:
- Enhancing fuel efficiency and reducing environmental impact
- Ensuring safety and structural integrity in harsh marine environments
- Protecting coastlines from erosion and preserving natural habitats
- Facilitating advancements in renewable energy production
Table:
Application | Industry | Benefit |
---|---|---|
Shipbuilding | Maritime | Improved vessel performance |
Offshore platforms | Oil & gas/renewable energy | Increased structural safety |
Coastal engineering | Civil engineering | Effective erosion control strategies |
The applications mentioned above are just a few examples that highlight the relevance of boundary layer study in floating hydrodynamics. As research continues to advance in this field, new possibilities emerge for enhancing efficiency, sustainability, and safety within various industries. The knowledge gained from studying the boundary layer provides invaluable insights that shape the future of floating structures.