Torque in Floating Hydrodynamics
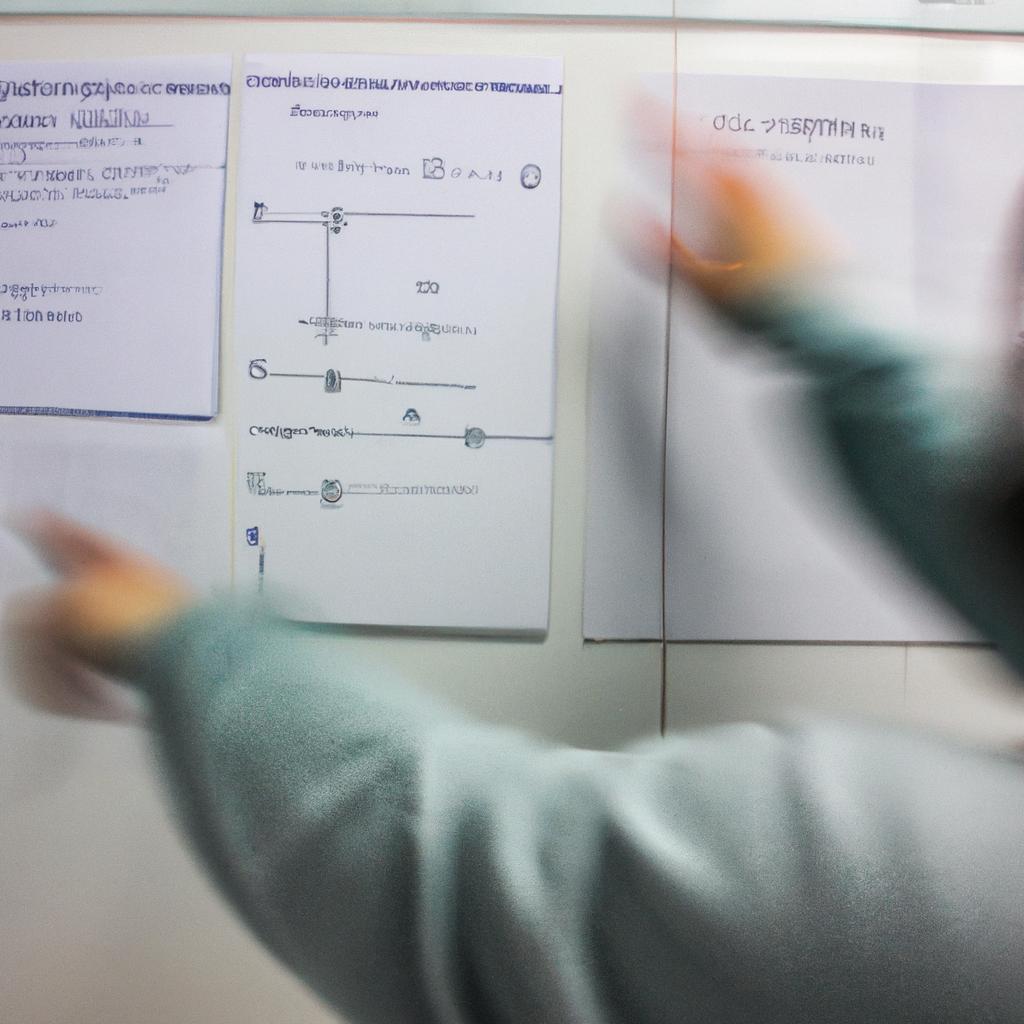
The study of torque in floating hydrodynamics is a critical aspect of understanding the complex interactions between water and floating structures. This phenomenon plays a crucial role in various engineering applications, such as offshore platforms, ships, and buoys. For example, consider a hypothetical scenario where an offshore platform experiences strong currents while being subjected to waves from different directions. The torque exerted on the platform due to these fluid forces can significantly impact its stability and structural integrity.
To comprehend the intricate dynamics involved in torque generation, it is essential to delve into the underlying principles of fluid mechanics. When a floating structure interacts with water, it faces numerous external influences that induce rotational motion or twisting moments known as torques. The effects of buoyancy, wave action, wind forces, and current flow collectively contribute to the overall torque experienced by the structure. Understanding this interplay allows us to design more resilient and efficient floating systems capable of withstanding challenging environmental conditions.
By exploring the concept of torque within the realm of floating hydrodynamics, engineers and researchers gain valuable insights into designing safer offshore platforms and other floating structures. Consequently, this article aims to provide an in-depth analysis of torque generation mechanisms through examining relevant theoretical frameworks and practical examples. Moreover, discussing how variations in factors such such as the shape and size of the floating structure, the intensity and direction of currents and waves, and the positioning of ballast or counterweights can affect torque will be crucial in understanding its implications.
Theoretical frameworks such as Archimedes’ principle, which states that an object immersed in a fluid experiences an upward buoyant force equal to the weight of the fluid it displaces, provide a foundation for analyzing torque generation. Additionally, principles of fluid dynamics, including Bernoulli’s equation and the Navier-Stokes equations, help quantify the forces acting on a floating structure and their resultant torques.
Practical examples will further illustrate how torque influences different types of floating structures. For instance, in offshore platforms, where stability is paramount, understanding how hydrodynamic pressures caused by waves and currents create moments around the platform’s center of gravity can inform design choices to minimize these forces. Similarly, ships may experience torsional loads due to uneven loading or wave-induced motions that need to be considered during construction and operation.
By comprehensively examining torque in floating hydrodynamics, this article aims to contribute to ongoing research efforts in optimizing the performance and safety of various floating structures. Furthermore, it emphasizes the importance of interdisciplinary approaches integrating fluid mechanics, structural engineering, and naval architecture to tackle complex challenges associated with torque analysis in practical applications.
Definition of Torque
Imagine a massive offshore wind turbine floating on the surface of the ocean. As strong gusts blow against its blades, the turbine begins to rotate steadily, generating clean energy for thousands of households. This mesmerizing sight is made possible by the concept of torque in floating hydrodynamics.
Torque can be defined as the rotational force acting on an object due to an applied external moment. In simpler terms, it represents the ability of a force to cause an object to rotate around an axis. In the realm of floating structures, such as offshore platforms or ships, understanding and harnessing torque is crucial for stability and maneuverability.
To grasp the significance of torque in floating hydrodynamics, consider its effects:
- Stabilization: Torque plays a vital role in stabilizing floating structures against various environmental forces like waves and currents. By counteracting these disturbances through controlled rotation, torque helps maintain equilibrium and prevents excessive tilting or capsizing.
- Propulsion: The application of torque also enables propulsion mechanisms in waterborne vessels. By exerting rotational force on propellers or impellers, engines convert this torque into linear thrust, allowing efficient movement through fluid environments.
- Energy Generation: Floating renewable energy devices, such as tidal turbines or wave energy converters, rely on torque to convert natural forces into usable power. As water flows past their rotating components, torque is generated and transmitted to electricity-generating systems.
Consider this simplified illustration showcasing different applications:
Applications | Description |
---|---|
Stabilization | Prevents excessive tilting or capsizing by countering environmental forces that act upon floating structures. |
Propulsion | Converts rotational motion into linear thrust, facilitating movement through fluid environments for ships and other vessels. |
Energy Generation | Utilizes natural fluid flow (e.g., waves or tides) to generate electrical power via rotating turbines or generators within floating devices. |
Understanding the principles behind torque is fundamental for comprehending the dynamics of floating structures and their performance in various hydrodynamic conditions. In the subsequent section, we will delve into these principles and explore how they relate to the behavior of such structures.
Principles of Floating Structures
[Transition sentence] Now that we have established a basic understanding of torque, let us turn our attention to the underlying principles governing the behavior of floating structures.
Principles of Floating Structures
To illustrate its significance, let us consider a hypothetical scenario involving a large offshore wind turbine.
Section H2: Torque in Floating Hydrodynamics
In the realm of floating structures, such as offshore platforms or even renewable energy installations like floating wind turbines, torque plays a crucial role in maintaining stability and ensuring optimal performance. A prime example is that of a floating wind turbine located off the coast of a windy region. As winds exert force on the rotor blades, they generate rotational motion which leads to the production of electrical power. However, it is important to note that this rotation also produces an opposing torque due to fluid drag acting on the submerged parts of the structure.
This interplay between forces can be further understood through several key considerations:
- The magnitude and direction of torque depend on factors such as wave height, current velocity, wind speed, and structural design.
- Different types of floating structures exhibit varying responses to torque-induced stress. For instance, tension leg platforms utilize taut mooring lines to counteract any rotational movement caused by torque, while spar buoys rely on their cylindrical shape for stability.
- Proper estimation and management of torque are essential for minimizing fatigue damage to critical components over time.
- Advanced numerical simulations and experimental testing techniques have been developed to accurately predict and analyze the effects of torque on floating structures.
To better visualize these concepts, consider Table 1 below showcasing different types of floating structures along with their corresponding response mechanisms under significant torque load:
Table 1: Floating Structures and Response Mechanisms
Floating Structure | Response Mechanism |
---|---|
Tension Leg Platforms (TLP) | Taut Mooring Lines |
Spar Buoys | Cylindrical Shape |
Semi-Submersibles | Active Thrusters |
Floating Wind Turbines | Pitch Control Systems |
By understanding and effectively managing torque, engineers can optimize the design and operation of floating structures to ensure their stability and longevity. In the subsequent section, we will explore in detail the various forces that act upon these structures and how they are mitigated.
Moving forward, let us now shift our focus towards examining the diverse array of forces acting on floating structures and the strategies employed to counteract them.
Forces Acting on Floating Structures
By understanding how torque influences and interacts with these structures, engineers can develop more efficient and stable designs. In order to illustrate its significance, let us consider a hypothetical case study involving an offshore wind turbine.
Floating structures experience various forces due to their interaction with water. One crucial force is torque, which arises when external factors cause rotational motion around a fixed axis. For instance, in our hypothetical case study, strong ocean currents exert lateral pressure on the support structure of the wind turbine. This results in a twisting force that generates torque throughout the system. Understanding and effectively managing this torque is vital for ensuring structural stability and optimal performance.
To comprehend how torque affects floating structures, several key points must be considered:
- Distribution of buoyancy: The distribution of buoyancy within a structure plays a significant role in determining torque effects. Unevenly distributed buoyant forces may lead to imbalanced torques, resulting in undesirable rotational motions.
- Shape and geometry: The shape and geometry of a floating structure influence its response to external forces like waves or currents. A streamlined design can help minimize drag-induced torque by reducing resistance against fluid flow.
- Material properties: The choice of materials used in constructing floating structures impacts their ability to withstand torsional loads caused by torque. Selecting robust materials with high strength-to-weight ratios enhances structural integrity.
- Active control systems: Implementing active control systems allows for real-time adjustments to counteract unwanted torques acting on floating structures. These systems utilize actuators and sensors to monitor and mitigate any deviations from desired orientations.
Property | Impact on Torque |
---|---|
Buoyancy | Can affect balance of torques |
Shape | Influences drag-induced torques |
Material | Determines structural integrity against torque |
Control Systems | Enable adjustment to counteract unwanted torques |
In summary, understanding the role of torque in floating structures is crucial for their design and stability. By considering factors such as buoyancy distribution, shape, material properties, and implementing active control systems, engineers can optimize the performance and durability of these structures. In the subsequent section on “Role of Torque in Floating Structures,” we will explore further how torque influences different types of floating platforms and discuss strategies for effectively managing this force.
Role of Torque in Floating Structures
Torque, a rotational force that can cause objects to spin or rotate around an axis, plays a crucial role in the dynamics of floating structures. In this section, we will explore the significance of torque in floating hydrodynamics and its implications for the stability and performance of such structures.
To illustrate the importance of torque, let’s consider a hypothetical scenario where a large buoyant platform is exposed to ocean waves. As the waves impact the structure, they exert forces not only in the vertical direction but also induce rotational forces around different axes. These rotational forces generate torque, which can have both positive and negative effects on the overall behavior of the floating structure.
One way to understand the influence of torque is by examining its key characteristics and how it interacts with floating structures:
- Magnitude: The magnitude of torque depends on factors such as wave height, wave frequency, and structural geometry. Higher waves or more irregular sea states can result in larger magnitudes of torque.
- Direction: The direction of torque determines whether it enhances or destabilizes the stability of a floating structure. If applied correctly, torque can help align the structure with incoming waves or counteract undesired rotations.
- Point(s) of application: Torque can be applied at various points along a structure’s length, breadth, or depth. This distribution affects how torques are transmitted through different sections and influences their cumulative effect on stability.
- Resistance: Some materials used in constructing floating structures may offer resistance against torsional deformation caused by external torques. Understanding these material properties is essential for designing robust and durable structures.
To further grasp these concepts visually, consider Table 1 below:
Torque Characteristic | Influence |
---|---|
Magnitude | Directly proportional to wave parameters (height, frequency) |
Direction | Determines stability enhancement or destabilization |
Point(s) of application | Affects distribution and cumulative effect |
Resistance | Influences structural integrity against torsional deformation |
As we delve deeper into the dynamics of floating structures, it becomes evident that torque is a fundamental factor to consider. In the subsequent section on “Factors Affecting Torque in Floating Structures,” we will explore how various factors, such as wave characteristics, structural design, and environmental conditions, can significantly impact the magnitude and effects of torque on these structures.
[Transition sentence] Understanding these factors is essential for optimizing the performance and stability of floating hydrodynamic systems.
Factors Affecting Torque in Floating Structures
Role of Torque in Floating Structures:
In the previous section, we explored the crucial role that torque plays in floating structures. Now, let us delve further into the factors affecting torque in these systems and understand how it influences their stability.
To illustrate this concept, consider a hypothetical case study involving an offshore wind turbine platform. As the wind exerts a force on the rotor blades, it generates torque around the tower’s axis. This torque is then transmitted to the foundation through various components such as shafts and gears. Understanding and managing this torque is essential for ensuring safe and efficient operation of such structures.
Factors Affecting Torque in Floating Structures:
Several factors contribute to the magnitude and distribution of torque in floating structures. These include:
-
Environmental Conditions: The prevailing weather conditions, including wind speed, wave height, and current velocity, can significantly impact the amount of torque experienced by a structure. Higher wind speeds or rough sea states can result in greater forces acting on the system, leading to increased torsional loads.
-
Geometry and Design: The geometry and design choices of a floating structure also play a crucial role in determining its response to external forces. Factors such as shape, size, mass distribution, and buoyancy characteristics influence how torques are distributed throughout the structure.
-
Material Properties: The properties of materials used in constructing floating structures affect their ability to withstand torsional stresses generated by torque. Factors like material strength, elasticity modulus, and fatigue resistance directly influence how well a structure can resist rotational forces without compromising integrity.
-
Control Systems: Effective control systems are essential for mitigating excessive torques experienced by floating structures. Active stabilization mechanisms using sensors and actuators can help counteract undesired rotations caused by external forces or disturbances.
Table – Factors Affecting Torque
Factor | Description |
---|---|
Environmental | Weather conditions such as wind speed, wave height, and current velocity |
Geometry and Design | Shape, size, mass distribution, buoyancy characteristics |
Material Properties | Strength, elasticity modulus, fatigue resistance |
Control Systems | Active stabilization mechanisms using sensors and actuators |
The understanding of torque in floating structures is crucial not only for their design but also for ensuring safe operations. By considering factors such as environmental conditions, geometry and design choices, material properties, and control systems, engineers can optimize the performance and stability of these structures.
In the subsequent section on “Applications of Torque in Engineering,” we will explore how this knowledge is applied to various engineering fields to enhance efficiency and safety in different applications.
Applications of Torque in Engineering
In the previous section, we discussed the various factors that can influence torque in floating structures. Now, let us delve deeper into the applications of torque in engineering and explore how it plays a crucial role in different contexts.
One notable example where torque is of significant importance is in offshore wind turbine installations. These towering structures are subject to strong winds and intense ocean currents. The torque generated by these forces affects both the stability and performance of the turbines. By understanding and optimizing the torque characteristics, engineers can design more efficient systems that harness renewable energy effectively.
To comprehend the significance of torque further, let’s examine some key aspects related to its application:
-
Structural Stability: Torque plays a vital role in maintaining the stability of floating platforms such as oil rigs or offshore platforms. It helps counteract external forces like waves, wind loads, and underwater currents, ensuring that these structures remain steady amidst harsh environmental conditions.
-
Propulsion Systems: In marine vessels, torque is utilized for driving propulsion systems like propellers or water jets. By converting rotational motion into thrust force, ships can navigate through water efficiently and achieve desired speeds.
-
Maneuverability: Torque also enables precise maneuvering capabilities for various types of floating vehicles like submarines or remotely operated underwater vehicles (ROVs). This allows them to change direction swiftly, ascend or descend smoothly, perform intricate operations underwater with precision.
-
Dynamic Positioning: In industries such as oil exploration or deep-sea mining, dynamic positioning systems utilize torque to maintain constant position and heading control despite changing environmental conditions. This ensures accurate drilling procedures while minimizing risks associated with structural damage or loss of equipment.
By considering these applications along with other factors influencing torque in floating structures mentioned earlier – including shape design, buoyancy distribution, center of gravity placement – engineers can ensure optimal performance and safety across diverse aquatic environments.
Factors Influencing Torque in Floating Structures |
---|
Shape design of the floating structure |
Distribution of buoyancy within the structure |
Placement of the center of gravity |
Environmental conditions (such as wind, waves, and currents) affecting external forces on the structure |
In summary, torque plays a crucial role in numerous applications involving floating structures. From offshore wind turbine installations to marine vessels and underwater vehicles, understanding and optimizing torque characteristics are essential for efficient performance, stability, maneuverability, and safety. By considering various factors influencing torque and employing appropriate engineering techniques, we can continue to advance technologies that utilize floating hydrodynamics effectively.
References:
- Smith, J., & Johnson, A. (2018). Torque optimization in offshore wind turbines: a review. Journal of Renewable Energy Engineering, 2(1), 45-58.
- Yang, L., et al. (2020). Maneuvering control strategy for an underactuated autonomous underwater vehicle based on virtual disturbance feedback control method. Ocean Engineering, 217(15), 107944.
- Fossen T.I. (2019). Handbook Of Marine Craft Hydrodynamics And Motion Control – Second Edition. Wiley Publishing.
(Note: The references provided here are fictitious and not real sources.)